Editing Out Pesticides
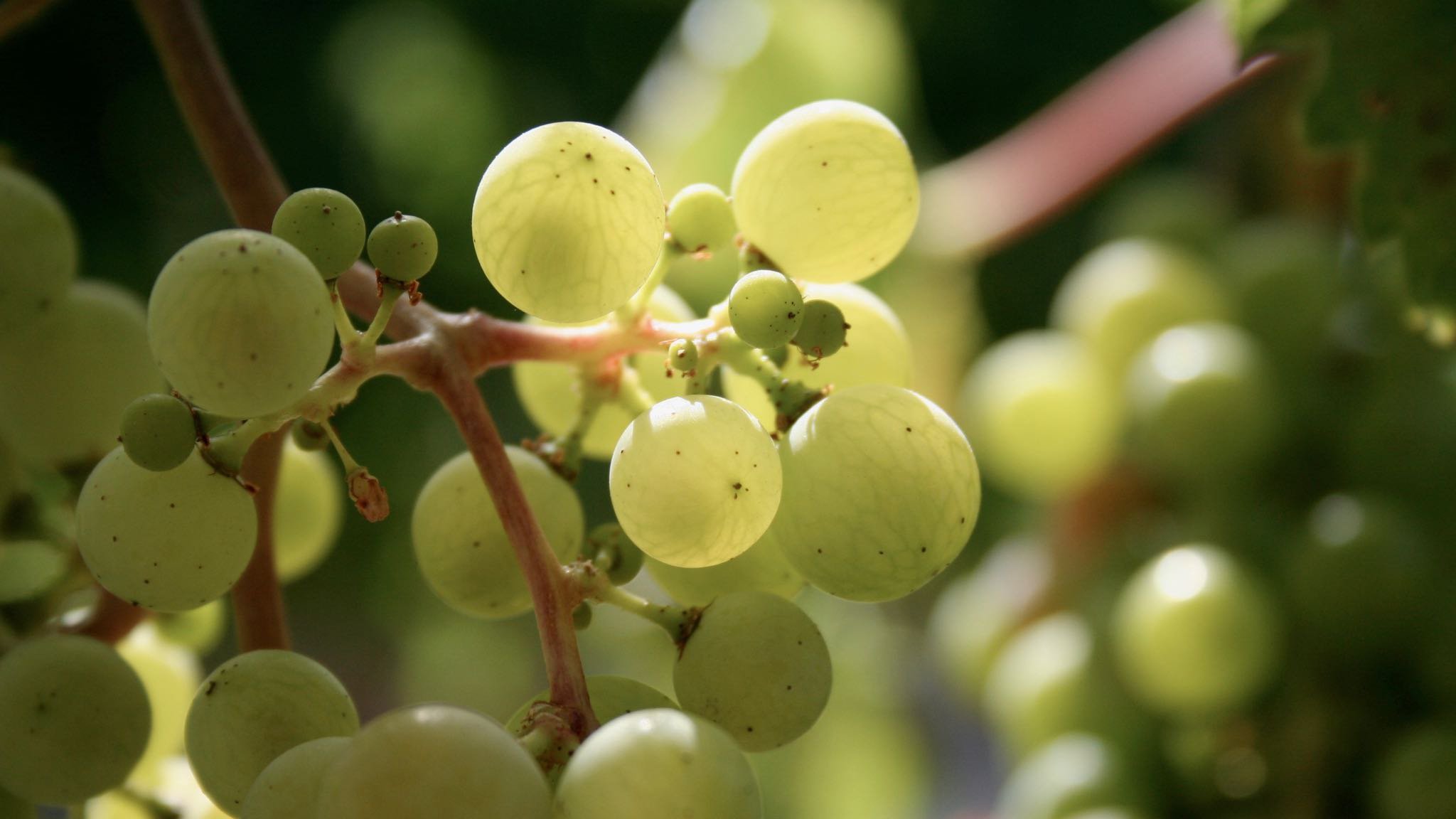
This summer, more than a million tons of chardonnay grapes are plumping on manicured vineyards around the world. The grapes make one of the most popular white wines, but their juicy fruit and luscious leaves are also targets for diseases such as downy mildew, a stubborn fungus-like parasite. If left unchecked, downy mildew coats grapes in white fuzz and strips the plant’s leaves, which means any surviving fruit won’t produce enough sugar to make good wine. Downy mildew is found practically anywhere wine grapes are grown, from California to Australia, New Zealand, and South Africa.
And New Jersey. The state isn’t widely known for wine; still, it’s seen a surge of wineries over the past few decades, partly because it’s close to key East Coast markets and because it mirrors the soil and, to a degree, the climate of France’s famed Bordeaux regions. One key difference is New Jersey’s hot and humid summers—ideal for downy mildew.
Some grape breeders try to avoid the pathogen by working with disease-resistant grapes, but it’s tricky for those who work with more well-known varieties. Chardonnay’s genetic consistency is great for preserving characteristics like taste and mouthfeel. The varietal’s genes trace back to the Middle Ages in eastern France, and they’re preserved, more or less, in all the chardonnays around the world today. But that consistency is less helpful when it comes to traits that resist pests and disease.
Drop a chardonnay vine into a new region where it didn’t co-evolve with the local pests, and it’s especially vulnerable. But breed a chardonnay with a hardier local variety, and you risk losing the very essence that makes it chardonnay. “There’s always some interest in new varieties with resistance,” says Dan Ward, director of the New Jersey Center for Wine Research and Education and a professor at Rutgers University. “But the fact is, winemakers want chardonnay or cabernet sauvignon, and consumers do, too.”
Since the market clamors for familiar varieties, New Jersey growers have been stuck with the vulnerable grapes, which require regular pesticide sprays for protection. But a new genetic tool called CRISPR may eventually offer an alternative: a chardonnay genetically edited to resist downy mildew.
“There’s always some interest in new varieties with resistance. But winemakers want chardonnay, and consumers do, too.”
The first hints of this grape are growing in small plastic boxes, their fragile roots piercing a clear nutrient gel, in the Rutgers lab of Rong Di , a molecular biologist and plant pathologist. Elsewhere, scientists are testing the technology on other crops to make disease-resistant staples such as rice and wheat. Others are also using CRISPR to manipulate and control insect pests directly.
“I remember a conversation with George Church in January of 2013, where we both looked at each other and said: This is going to be huge,” says Jennifer Doudna , a biochemist at the University of California, Berkeley and one of the scientists who made some of the most publicized CRISPR discoveries, along with Emmanuelle Charpentier of the Max Planck Institute for Infection Biology and two other teams led by Church , a geneticist at Harvard Medical School, and Feng Zhang , a bioengineer at the Broad Institute of Harvard and MIT. The potential, she adds, includes “the good, possibly the bad, the public interest, the hype, the hope” and all of the other uses of the technology “that could positively impact mankind.”
“I think that has really been borne out in what is going on right now,” Doudna says. “And with respect to agriculture, I think we’re just at the tip of the iceberg, frankly.”
Controlling pesticides
Today, winegrowers struggle to keep downy mildew at bay. They use cultural practices to keep the pathogen from gaining a foothold, such as pruning, which aerates the remaining leaves before the mildew can germinate. But pruning isn’t enough. Growers also must run regular reconnaissance through their rows to catch the early signs. If the growers see the telltale yellow blotches that spatter across the leaves like drops of oil, or if weather patterns and other signs predict an outbreak, they have to treat their fields with fungicides.
The battle between wine grapes and downy mildew is so old that the disease was the target of the world’s first widely used fungicide, which was discovered in France in the 1880s. As the story goes, Bordeaux winegrowers would spray copper sulfate and lime on vines that abutted roads because the blue mixture’s bitter taste kept travelers from eating the grapes. French botanist and mycologist Pierre-Marie-Alexis Millardet noticed these plants were free from downy mildew and began testing the concoction to control the disease across local vineyards. Eventually, farmers worldwide were spraying the Bordeaux Mixture on grapes, potatoes, peaches, and more.
Today, winegrowers still use copper sprays on vineyards, as well as modern synthetic fungicides. Copper—used by both conventional and organic growers—remains in soil for a relatively long time and can be toxic to fish, aquatic invertebrates such as shrimp and crabs, bees, earthworms, and more. And although modern synthetic fungicides common on vineyards today, such as azoxystrobin and captan, have relatively low toxicity to mammals, birds, and bees, they are dangerous to fish and other aquatic animals and can persist in soil or water. New Jersey growers spray their vineyard six to 12 times a season to treat downy mildew and other diseases.
While growers may limit hazards by spraying sparingly, there are other issues with the chemicals. Many downy mildew strains have grown resistant to fungicides—an inevitable result because of evolution—and the options for replacement are dwindling. The fungicides are expensive, too, especially when compared with similar crops. According to Ward’s estimates, it costs $264 to $528 per acre to control downy mildew in the state. By comparison, field corn may require just a few pesticide sprays a season at a lower cost per acre .
These environmental and economic pressures are common across agriculture. Pesticide resistance is a growing problem among insects, weeds, and diseases worldwide, and the discovery and development of new pesticides is slowing, partly due to high costs—research and development on a single active ingredient runs around $286 million over 11 years. Climate change is driving many pests beyond their usual home ranges, too. And our population will continue to grow—we’ll reach an estimated 9.7 billion by 2050 —potentially taxing our global food system.
Clearly, we’ll need new ways to control pests.
For grapes, Di’s work with CRISPR may help. CRISPR is a gene editing technique often compared to a biological word processor. If the chemicals that make up DNA are an alphabet, those letters, in turn, spell out the instructions for the particular characteristics of a plant, animal, or any other organism. Gene editing allows for precise editing to delete, add, or replace a specific set of letters, which can change the physical characteristics. Di is using the technique to shut off the genes that make chardonnay vulnerable to downy mildew, which will make the plant hardier against the disease. The variety is Dijon chardonnay 76—she’s nicknamed the edited grapes NJ 76.
“There are a host of factors the pest uses to attach to the plant,” she says. “With CRISPR, we hope we can cut off the interaction with the host and downy mildew so the fungus can be stopped.”
Di’s says her first results may be complete later this year. But whether wine connoisseurs will prefer a gene-edited chardonnay or a conventionally bred no-name blend is also uncertain. “The answer to that will be decisive in whether these succeed in the real world, in the market,” Ward says. The technology could produce the qualities growers want, but “there’s a bunch of factors I can’t predict.”
The fate is also uncertain for all CRISPR’d agriculture, which depends not only on whether scientists can pull off their technological feats, but also how regulators and the public ultimately view gene editing. The development of these crops runs in parallel to a complicated discussion over semantics, regulation, and risk perception. And as with all new technologies, there are tricky and yet-unanswered questions: What are the benefits? What are the risks? And which outweighs the other?
Bacterial origins
Gene editing isn’t new. The earliest tools have been around for 20 years, and for the last five or so, scientists have used techniques with clunky names such as zinc-finger nucleases and transcription activator-like effector nucleases (TALENs) to edit the DNA of plants and animals. If CRISPR is like a modern word processor, these older technologies are more like editing a document with a Commodore 64.
In the wild, CRISPR is essentially a genetic immune system found in a wide range of bacteria species. When viruses infect the bacteria, the system identifies the viral DNA and chops it up. The key difference from older techniques is how they are built in the lab.
The molecular machinery that helps zinc fingers and TALENs find DNA requires many steps and biological parts, and scientists generally have to make the tools from scratch. Scientists can set CRISPR up more easily and quickly in the lab because its components are simpler to build. Many pieces can even be ordered fully-assembled from biological supply companies.
The implications for the new technology have only become clear in the last few years. The initial research—and ethical considerations—focused on medical applications. But the system is relevant to anything with DNA, which means it can be used to alter the genes of any living thing—including crops and livestock.
The excitement for the technology is hard to miss. Di, for example, is typically soft-spoken, but when the discovery of gene editing comes up her voice jumps about an octave and her hand gestures get bigger and looser. She recalls the first time she heard about TALENs, from a colleague at a party. “Oh my god. I spent three days and read all the papers on TALENs and plants,” she says. “I said, ‘This is genius! This is great!’ ” She ordered the relevant materials and applied for a grant to use TALENs on downy mildew in grapes.
Then CRISPR hit scientific journals, and she changed course. “Immediately, I switched, because I saw the advantage of CRISPR.”
Beyond grapes
While disease-resistant chardonnay solves an economic and marketing problem, it isn’t exactly a staple food that’s going to help feed the world. But elsewhere, researchers are using gene editing to make everyday crops disease-resistant. For example, scientists at Iowa State University are creating rice that is resistant to bacterial blight and rice blast, two of the cereal’s most common and devastating diseases. Pennsylvania State University scientists are also working on blast-resistant rice and have plans to make blight-resistant potatoes. And a team from the Chinese Academy of Sciences has made wheat in the lab that is resistant to powdery mildew.
In February 2016, the United States Department of Agriculture awarded a $4 million grant to a team from the University of California, Riverside, the University of Florida, UC Davis, and Texas A&M to make sweet oranges that are resistant to citrus greening, a bacterial disease. Citrus greening is spread by a small insect called the Asian citrus psyllid. The insect has overtaken orchards in Florida and may do the same in California. There is no cure; the only way control it is to prevent infection in the first place, usually by using insecticide against the psyllids.
At Cornell University, scientists are taking a different approach to fighting pests in rice. While the researchers are working on specific pathogens, they also want to uncover the genetic underpinnings of general vulnerability to disease and then tweak that with gene editing.
“This is what we call basal disease resistance—overall plant immunity,” says Adam Bogdanove , a plant pathologist on the Cornell team. “Some of our friends never get sick. Some of our friends get sick all the time, you know? It’s just how robust their immune system is, and that analogy applies to plants as well.”
“The ideal approach would be to eradicate the pest.”
Still other researchers are taking a different tack. In addition to small DNA edits, CRISPR can be used with another tool called a gene drive. Gene drives manipulate inheritance by forcing a trait into virtually all offspring, where they would otherwise have a 50-50 chance of inheriting it. This could allow researchers to genetically engineer entire populations of insects in the wild.
So far, most work with gene drives focuses on decimating mosquito populations to curb human diseases like Zika or malaria. But some groups hope to apply the technology to agricultural pests. Two separate teams at North Carolina State University and the University of California, Riverside , for example, are exploring gene drives for Drosophila suzukii , the spotted winged fruit fly. This pest lays its eggs under the thin skins of ripening cherries, strawberries, and blueberries, and its larvae hatch to munch the sweet flesh.
“We’re taking multiple approaches. The ideal approach would be to eradicate the pest—develop a catalytic gene drive system that you could release into a population that can spread invasively,” says Omar Akbari, a molecular biologist at UC Riverside. “And as it spreads, the population declines without the use of insecticides.”
The patent problem
All of these researchers are in university labs, and their work is publicly funded. For example, the USDA supports the Rutgers, NCSU, Penn State, and Iowa State projects, as is the UC Riverside fruit fly project (although the latter also has some money from the California Cherry Board). And the Cornell researchers received a competitive grant from the National Science Foundation.
This is partly because of an ongoing intellectual property dispute between the groups that discovered CRISPR, with the University of California and the University of Vienna on one side and the Broad Institute, MIT, and Harvard on the other. Whichever group gets the patents will determine how the technology is licensed to other companies.
“The university labs have this really wonderful position where all they need to do is science,” says Sara Olson, an analyst at Lux Research who specializes in emerging agricultural technology. Companies, on the other hand, have to worry about licensing and selling products.
But some companies are forging ahead anyway. Last October, Caribou Biosciences , co-founded by Doudna, announced it would license its version of CRISPR to DuPont. In April, DuPont Pioneer announced its first commercial CRISPR crop, which it expects to be on the market within five years. Caribou also plans to develop its own agricultural products.
The biotech company Cibus has recently gene-edited crops with a trait already familiar to agriculture: herbicide-tolerance, which means they can withstand pesticide sprays intended for nearby weeds. Cibus sells sulfonylurea-tolerant canola made with a gene editing technique the company calls the rapid trait development system, and it plans to launch glyphosate-tolerant flax made, in part, with CRISPR in 2019.
Cibus’s approach shows that gene editing won’t always necessarily reduce pesticide use. Over the past few decades, the popularity of glyphosate-tolerant crop made with older techniques grew may have helped drive herbicide-resistance in weeds.
Resistance is inevitable. If farmers repeatedly use a single approach to control a pest, such as a synthetic pesticide, they’ll kill most of the pests, but a few individuals will have a trait that helps them survive and will pass their hardy DNA to the next generation. Some weeds produce high numbers of seeds, increasing the odds that resistance will spread. For example, an individual palmer amaranth plant—a notorious weed that has grown resistant to glyphosate across the United States—produces an estimated 500,000 seeds.
Glyphosate resistant weeds may lead farmers to revert back to older and more toxic herbicides. If farmers over-use Cibus’s technology, the crops could see the same fate.
“Using a different technology to get the same type of herbicide resistance in a crop plant would not matter in my opinion,” says Allison Snow, a plant ecologist at the Ohio State University. “What matters is whether glyphosate use and dosage increases over time and what effect this has on selection for weeds that become resistant to increasing dosages of this herbicide.”
The branding question
The gene-edited crops and insects these scientists are creating face an uncertain future, which depends, in part, on how convincingly they brand the technology.
At the heart of this struggle are genetically modified organisms, or GMOs. While the term GMO is slippery , it generally refers to a food that has been altered using recombinant DNA, which inserts genetic material—sometimes from a different species, called a transgene. For example, scientists use plant pathogens to shuttle this genetic information inside the plant cells, which naturally invade the cells. Skeptics have criticized the technology since its inception, and there are ongoing debates in the U.S. on whether GMO foods should be labeled. For this reason, it’s no wonder companies like Cibus call their gene-edited crops “non-GMO.”
In conversations stressing the differences between GMOs and gene editing, three points often arise. First, while scientists can’t control where the genetic material ends up in a GMO, gene editing is programmable and relatively precise. Second, GMOs made with plant viruses will always contain traces of the DNA from those viruses, while plants made with CRISPR may not. Third, GMOs often contain genetic material from a different species—for example, a crop that can make bacterial toxins that help protect it against certain pest insects—while CRISPR allows for smaller tweaks to existing genes within the organism itself.
In some cases, a plant made with CRISPR will be radically different from a GMO, while in others it may be less so.
But this is where things get tricky: While CRISPR crops might not contain foreign DNA, the process may still require it. Some scientists still use plant pathogens to insert CRISPR into their crops. Others, like Di, use genetic material from cauliflower mosaic virus, a plant pathogen, which helps make sure the CRISPR material is turned on inside the target plant. In most cases, the foreign DNA from these plant pathogens can eventually be removed through additional breeding.
“In the next generation, we can lose the transgene, returning to the mutation we want,” Di says. “So the plants can be considered not transgenic.”
And while CRISPR can be used to make small tweaks, it can also be used to insert foreign genes from other species. So in some cases a plant or animal made with CRISPR will be radically different from a GMO, while in others it may be less so.
Critics suggest this fight over semantics is a way for scientists to have their cake made with gene-edited flour and eat it too. Biotech advocates have argued for years that all crops are technically GMOs, says Glenn Stone, an anthropologist who specializes in environmental studies at Washington University in St. Louis. Even conventional breeding involves humans tinkering with genes in order to produce favorable traits, which means most plants or animals we consume have been artificially altered.
Many of those same scientists who say all crops are GMOs now argue that a crop made by genetic editing is decidedly not a GMO, Stone says. “So corn seeds planted by ancient Mexicans are GMOs, but seeds that have had their DNA cleaved by nucleases and then had donor DNA added are not GMOs,” he says. “I’m reminded of Bizarro World in Superman comics.”
The definitions serve a purpose. “They clearly want to avoid the stigma of what some people call ‘the Monsanto problem,’ ” Stone adds, referring the world’s biggest producer of GMO seeds, as well a common target for people who oppose GMOs.
“Public opinion hasn’t started to take shape yet, but I suspect the names will matter quite a lot,” he says. “The names will probably affect whether people transfer their entrenched objections to ‘conventional GMOs’ to gene-edited organisms.”
The regulatory hurdles
Gene editing faces regulatory uncertainties, too. Back in the 1980s, U.S. policymakers decided to use the Coordinated Framework for Regulation of Biotechnology to regulate foods made with biotechnology through existing laws. Different aspects of regulation fell to three groups: the USDA, which is concerned with the effect on other plants and animals, particularly whether a biotech crop acts as a plant pest; the Environmental Protection Agency, which is responsible for any genetically-engineered crops that relate to pesticide use; and the Food and Drug Administration, which is responsible for food safety.
Critics argue that genetic engineering has evolved too rapidly for these regulations to keep up. Gene editing technology such as CRISPR are stretching the regulatory system even more. “We’re sort of fitting—in some instances—square pegs into round holes,” says Greg Jaffe, biotechnology director for the Center for Science in the Public Interest. “We’re using old statutes that didn’t anticipate such technology or products, and doesn’t directly on point relate to those.”
Over the past few years, the USDA has said it wouldn’t regulate several gene-edited crops because they don’t trigger its requirements for oversight. While these particular cases don’t pose an obvious looming threat—the first CRISPR food to get a pass from the agency is a mushroom that browns more slowly than normal thanks to a small genetic tweak—the system won’t necessarily catch cases that pose real concerns.
That may change. In July 2015 , the Obama administration called for an update on the coordinated framework. According to the Office of Science and Technology Policy, the proposed language will be available for public comment this summer. The USDA is also reviewing its regulatory process. And the National Academy of Sciences asked a team of experts to consider new biotechnology advances and products that may emerge over the next five to ten years. Their report, intended to help regulators prepare, will publish later this year.
“There are still bound to be concerns about risk.”
Industry and academic scientists who find the current regulatory process unpredictable and burdensome hope for a streamlined system with a clearer path to market. But just like any technology, gene editing has the “bright side and the dark side,” says Jennifer Kuzma, a professor of science and technology policy and co-director of the Genetic Engineering and Society Center at North Carolina State University. “I don’t think any technology escapes that. On the positive end of that spectrum is that the ease of CRISPR may democratize biotech for smaller companies, nonprofits, and academic groups.”
There may also be new benefits, she adds, including products designed for social good, like environmental remediation, biofuels, and replacements for pesticides. As for the dark side: “There are still bound to be concerns about risk depending on the product and the context, as well as the molecular technique,” Kuzma says. “I’m not sure it gets a free pass on all safety issues.”
One potential risk is an off-target mutation, where the tech makes a genetic change at an unintended spot. If gene editing is like word processing, off-target mutations are like deleting or replacing the wrong word. In some cases, off-target changes could amount to nothing. Critics worry that in extreme worst-case scenarios, these editing mistakes may lead to a plant that produces unexpected allergens or toxins.
Experts don’t agree on the risks. A 2014 , study from Harvard University found that off-target mutations were rare in human cells altered with TALENs and CRISPR, although other researchers have expressed concern that not enough is known to correctly predict the events. Similar research in plants is limited. Some work suggests that off-target mutations are low in the model plant Arabidopsis . And a team at Michigan State University has an ongoing project comparing off-target hits in potatoes made with CRISPR, TALENs, and older breeding techniques.
Then there is the matter of using gene drives to alter entire populations of insects in the wild, and whether those alterations will be controllable. There may be a solution, albeit an imperfect one. In 2014, George Church and his colleagues proposed a safety mechanism : a second gene drive that would reverse the effects of the first gene drive. That may control the population, but as the authors note, “any ecological changes caused in the interim would not necessarily be reversed.”
Another concern is gene flow, in which genes move between different populations. This may be a particular issue for crops. In the 1990s, evolutionary geneticist Norman Ellstrand pointed out that because plants could breed at relatively far physical distances—for some species, swapping DNA with plants that are miles away—biotech crops might mate with their wild relatives.
The worry, for example, is whether a crop engineered to withstand diseases or environmental stress could cross with a weedy wild counterpart, making that weed even harder to control. So far, the most common GMO crops in the U.S., such as corn, don’t have wild counterparts with which to swap genes. But if gene editing makes it faster and cheaper to bring crops to market, it may be used on varieties that do.
It may also be harder for scientists to detect whether these genes have moved to wild populations, Ellstrand adds, if a gene-edited plant has only subtle genetic changes and doesn’t contain traces of the biological tools that created it.
“Gene flow is going to get things eventually into a population where you might not think the gene will end up,” says Ellstrand, who is now a professor of genetics at UC Riverside. “And if you don’t care that it’s in that plant population, it’s not a big deal. But if you do care, it’s a human value issue.”
Are we ready?
Ultimately, whether we use CRISPR in agriculture comes down to a handful of questions: Which is better, controlling plant diseases and pests with pesticides, or creating new varietals like a CRISPR’d, mildew-resistant grape? Which is more economical? And do chemical pesticides or gene-edited crops pose more risk to people and the environment?
It depends, in part, on how individual products are made and used and whether they affect unintended targets in the surrounding environment. But experts suggest the new technology is worth exploring.
“We should use all the different tools at our disposal to try to reduce the amount of synthetic chemicals that we use to produce the food that we eat,” Jaffe says. “Whether that’s using genetic engineering, DNA editing techniques, or integrated pest management, there are a lot of tools that can be used separately or in combination to help reduce the environmental footprint for agriculture. To the extent that a technique is safe, we should be open to using that technology.”
Back at Rutgers, Di steps down the hall to visit her fledgling NJ 76 plants. She swings open a heavy door to a small, brightly lit room lined with metal racks. Rows upon rows of clear boxes hold tiny green plants in various stages of development—strawberries, asparagus, and NJ 76. Di bends down to open a cardboard box marked “GRAPE” in black marker, revealing stacks of petri dishes cradling dabs of NJ 76 starter cells, which she’ll use to grow new plants.
Under a microscope, the grape cells look like dollops of lumpy porridge. “Each one of these little grains,” she says, “can potentially grow into a plant.”
And if the gene editing process works, and the world gets ready, each of those grains may contain the new genetic material to grow generations of downy-mildew-resistant, pesticide-free grapes.
Photo credits: Jon Rawlinson/Flickr (CC BY) , Henry Hemming/Flickr (CC BY) , Brooke Borel
Correction: Previously, this article stated that Cibus sells glyphosate-tolerant canola. The company sells sulfonylurea-tolerant canola.