The uplifting science of how dandelion seeds stay aloft
Two research teams went into the weeds to quantify the magic behind the flight of the dandelion seed.
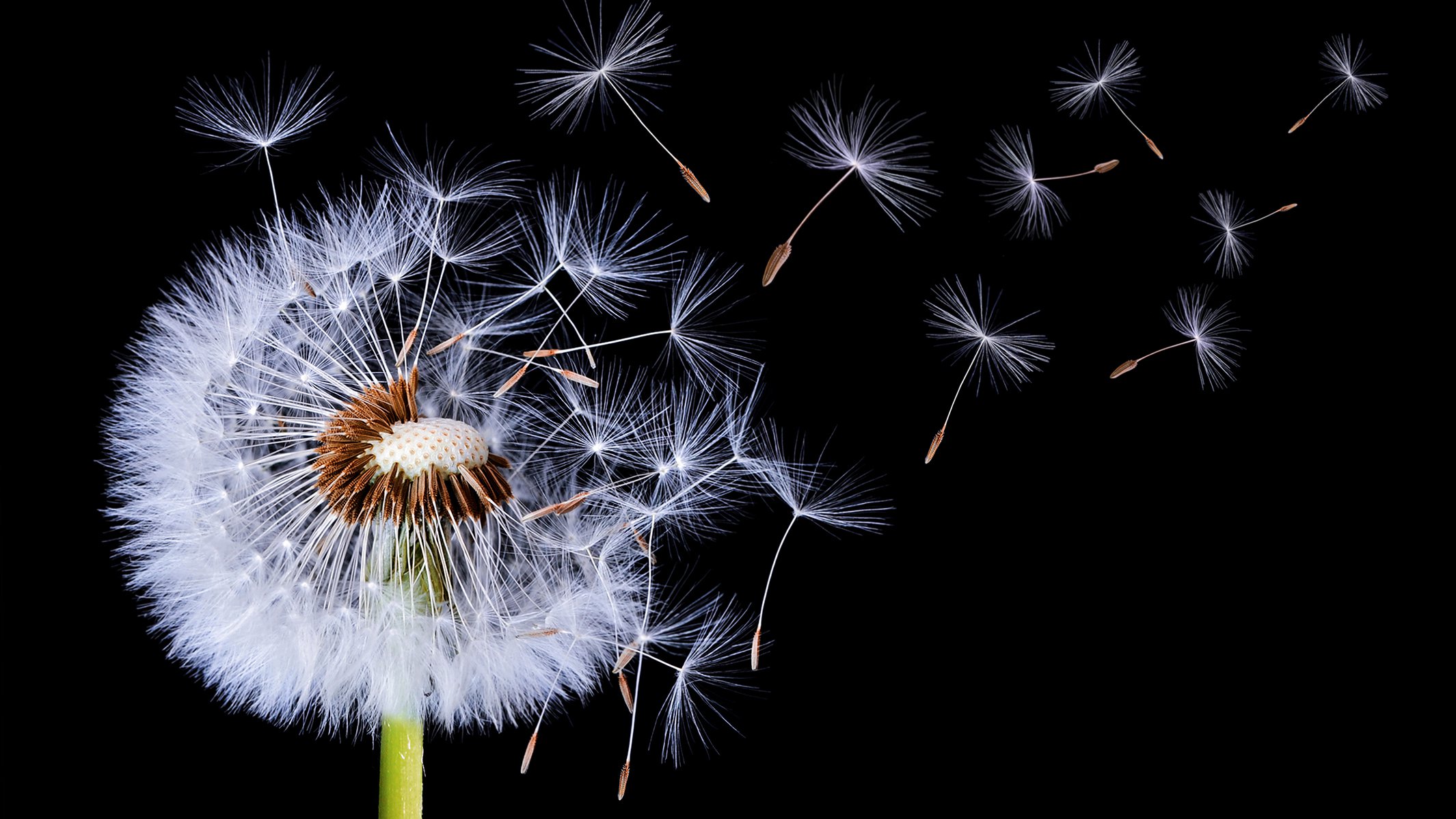
With two recent studies, the physics behind dandelion seed dispersal is now taking flight. Image Credit: Bess Hamitii, Shutterstock
At some point or another, most of us have played travel agent to a dandelion. Blowing on their tufted tops is a childhood rite of passage for many, and a simple puff of air is all it takes to send the seeds of these wayfaring weeds wafting into the breeze.
But even when our lungs are the source of the initial gust, we humans can’t take all the credit for dandelion fight. Thanks to evolution’s incessant tinkering, these seeds have become some of nature’s deftest dispersers, tumbling inches, feet, sometimes miles from their original source. Now, two recent studies reveal the physics behind their fanciful flight.
In the wake of their golden days of flowering, the tops of dandelion plants mature into dozens of flight-ready seeds, each tethered to its own personal parachute. But what crowns these seeds aren’t the billowing cloth contraptions we’re used to. Rather, they’re tufts of haphazard fluff, each made up of about 100 individual bristles that fan out into a sparse, delicate plume called a pappus.
To suss out how these strange little structures keep seeds aloft, a team of University of Edinburgh engineers led by Cathal Cummins went into the weeds. Using a tiny, vertical wind tunnel that suspended seeds at a fixed height, simulating flight, the researchers were able to observe how air interacted with the pappus’ filaments.
The trick behind the seeds’ airborne acrobatics, it turned out, was less about the bristles themselves than the gaps between them. High-speed cameras showed that, after air flowed up and around the pappus, it was quickly rerouted into the center of a swirling, elongated whirlpool called a vortex ring that pushed the air back down toward the top of the pappus, where it ascended once more. This fast-moving funnel was so good at recycling air that it generated an ever-present pocket of low pressure just above the seed, sucking it upward into an easy, breezy cruise—and delaying its inevitable descent.

Fun fact: Each unit of a dandelion is actually home to hundreds of individual flowers clustered together. Because dandelions can reproduce both sexually (through pollination) and asexually, every seed emerging from a dandelion’s head can have a different genetic makeup. Image Credit: Theo Crazzolara, flickr
Dandelions are by no means the first organisms (or even the first plants) that have been shown to DIY their own vortex rings to achieve liftoff. But as Cummins and his colleagues reported last October in the journal Nature, their pappuses have an especially aerodynamic structure, with bristles so thin that they take up just 10 percent of the total space in the seed’s frizzy updo. This gives the pappus a lot of porosity—a measure of its relative “emptiness,” explains study author Madeleine Seale, who specializes in plant biomechanics.
And dandelion seeds, it would seem, have a knack for turning nothing into a whole lotta something. Compared to a solid, parachute-shaped structure of about the same size, the pappus is actually four times as efficient at creating drag, the force that counteracts gravity to keep the seed airborne, the researchers found.
There was a second effect, too: By generating super-efficient drag, the pappus’ porosity also has the perk of keeping the seed’s flight stable.
When upward-bound air travels up and around a falling object, it can create a sort of wobble—a bit like a flag flapping in a gust of wind, just on a much smaller scale. But because the pappus is porous, some air flows through it instead, streaming between the bristles of the seed’s thin toupee. The more porous the parachute, the smoother its flight.
What wasn’t quite clear, however, was if the seeds’ stability was affected by the number of filaments in each pappus. That’s where the second paper, published today in the journal Physical Review Fluids, comes in. Taking a theoretical approach, a team led by fluid dynamicist François Gallaire of the Swiss Federal Institute of Technology Lausanne devised a mathematical model of the super-steady nature of dandelion vortex rings.
The results revealed a fragile balancing act between two opposing forces. The fewer filaments atop a seed, the higher its porosity, and thus stability—good news for a skyfaring seed. But stability also comes at the cost of drag, which increases as the number of bristles goes up. A fair compromise, the researchers found, is for the seeds to hit a sweet spot around 100 bristles per pappus. This figure matched up with what’s found on real-life dandelions, Gallaire says. There’s some wiggle room, but at lower numbers, the seed would have trouble staying afloat; too much higher, and things might start to get shaky.
“It is super cool that dandelions and this mathematical model came up with the same number,” says Joan Edwards, a plant biologist at Williams College who was not involved in either study. “Adaptation is one of the hardest questions to answer in evolutionary biology...but now, we can theoretically explain why a pappus has this number of filaments, and that’s really exciting.”
But the theoretical model does more than add credence the first paper’s experimentally-driven results, Seale says. “With a model, they can explore things we can’t explore experimentally very easily...including how these structures can be used in new and different ways,” she says.
For instance, plugging different numbers into the Swiss team’s equations could aid the design of better microdelivery systems, says Siobhan Braybrook, who studies plant form and function at the University of California, Los Angeles, but was not involved in either study. Someday, pappus-like structures could play a role in tasks like airdropping fertilizer or transporting teeny robots.
One thing that’s probably not in our future, though, is a dandelion-inspired parachute for people or their parcels, Braybrook adds. We humans (and most of the things we buy) are far too big and bulky for the same physical principles to apply. “For this design to be relevant...you need to talk about objects that are incredibly light or incredibly small,” she says.

The dandelion's sparse, tufted pappus creates a swirling pocket of air called a vortex ring in the space above it. The pappus is mostly empty space, but the 100 or so filaments that comprise it hit a "sweet spot" between creating just enough drag to keep it aloft, while maintaing the seed's stability. Image Credit: Courtesy of Cathal Cummins, Madeleine Seale, Enrico Mastropaolo, Ignazio Maria Viola, and Naomi Nakayama
Unless, that is, we fiddle with one pesky constraint: gravity itself. “If we were on a different planet...we might need to come up with different ways of dealing with drag,” Braybrook says. “In that kind of situation, [this model] could be incredibly relevant.”
We’re not quite ready to deploy those tactics just yet. In the meantime, there are still exciting opportunities to study some marvels of innovation right here on Earth: Many other plants spread their seed by wind; even some animals have evolved specialized structures that help them navigate through air or water.
As research on these organisms and more continues to take flight, a one-size-fits-all equation probably won’t apply. But maybe that’s part of the beauty of this dazzlingly simple system.
“Nature has optimized something so beautiful,” Braybrook says. “For us, it’s only as we start to develop [new technologies] that we start thinking about these problems...but nature’s already done the work. We have the best simulations ever in the products of evolution.”