The Evolution of the Bioinspired Robot
If you're not prepared for the sight of them, Howie Choset's robot snakes can make you jump. They slither between chairs and tables in his Carnegie Mellon lab, rear their heads to peer into crevices, and inch their way along pipes on the ceiling. When dropped into a pond, they switch over to sea serpent-mode, twisting tightly as they skim through the water. Like an ophidiophobe's worst nightmare, they will even wrap around your leg and inch their way up toward your groin, if given the chance. "The benefit of the snake is that it's able to reliably get to locations that people and machines can't," Choset says.
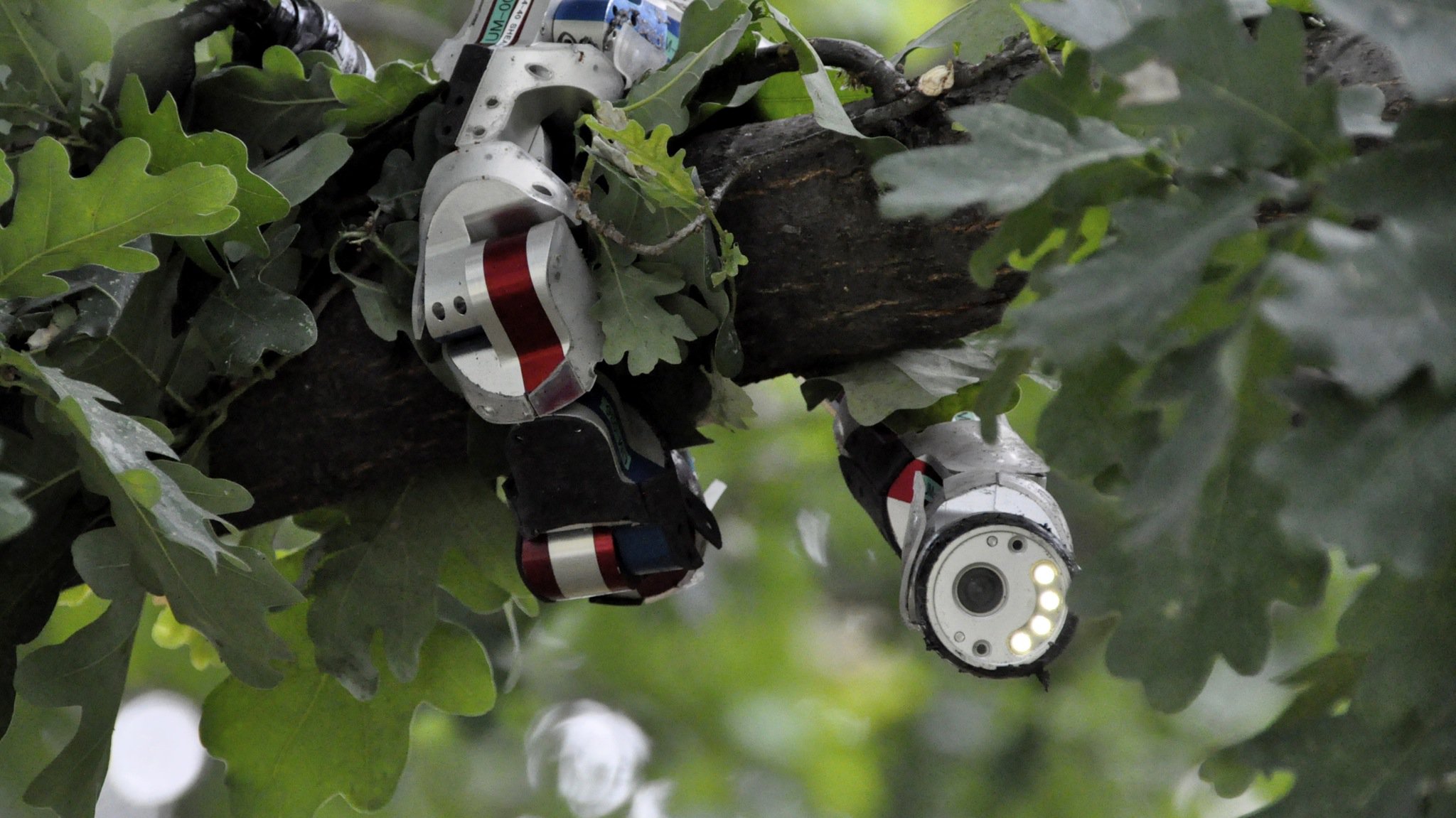
If you’re not prepared for the sight of them, Howie Choset’s robot snakes can make you jump. They slither between chairs and tables in his Carnegie Mellon lab, rear their heads to peer into crevices, and inch their way along pipes on the ceiling. When dropped into a pond, they switch over to sea serpent-mode, twisting tightly as they skim through the water. Like an ophidiophobe’s worst nightmare, they will even wrap around your leg and inch their way up toward your groin, if given the chance. “The benefit of the snake is that it’s able to reliably get to locations that people and machines can’t,” Choset says.
These undulating machines may move like living snakes, but they can do things no ordinary serpent can. They can be launched through the air toward a tree or pole and, upon contact, will swiftly wrap around it, unharmed, in a tight coil. Cameras on their heads transmit footage back to their operators. Their length can be quickly changed by adding or removing joints, and those same segments endow them with a greater range of motion than any real-world snake could manage. That’s in part because snakes are burdened with over 100 million years of evolutionary baggage.
But if nature isn’t perfect, neither are robots. Robots may be devoid of pain, endowed with skeletons of steel, easily modified, and adroitly controlled by humans, yet nature, with its versatility and adaptability, continues to outperform the most high-tech bioinspired machines available today.
Nothing makes that point more clearly than Choset’s snakes, which despite their prowess, life-like grace, and extraordinary abilities, were utterly helpless when confronted with an ordinary mound of sand.
Superlative Start
If you were to trace the origins of bioinspired robotics, a good place to start would be the office of biologist Robert Full, circa 1986. Then, Full was a new professor at the University of California, Berkeley, and he had a problem. He was hoping to undertake a new study on cockroaches—not the most beloved creature in the animal kingdom—and like so many researchers, he desperately needed funding. Sifting through page after page of rules and funding requirements, he found what seemed like a loophole. If he teamed up with industry researchers, he could get more grant money.
By chance, he soon met robotics pioneers Marc Raibert and Rodney Brooks. (Raibert would later found Boston Dynamics, arguably one of the most advanced robotics company in the world, while Brooks would go on to found iRobot, the company behind Roomba.) After talking, the trio decided to join forces. Full got to work on what he assumed was a flamboyantly embellished grant proposal. “I said, ‘We’re going to be able to create the fastest robots that can climb and go places that no other robots can go,’ ” he recalls. “I felt so guilty, thinking ‘I’m totally making this up!’ ”
They ended up getting the grant.
Full, Raibert, and Brooks’s initial collaboration—which stressed the importance of rudimentary control and mechanics in insect-like legged robots—proved much more than a one-off. “When I look back, I laugh,” Full says. “We wound up doing so much more.” As it turned out, the three were the vanguard of an entirely new field referred to by any number of names—biomutalism, locomotion systems science, computational neuromechanics, or robiophysics. Despite the diversity of monikers, its practitioners all have one thing in common—they team up with other like-minded, but differently trained, scientists to create autonomous and artificial approximations of living systems.
As biologists have lifted the veil from one biological enigma after another, they have been welcomed into robotics.
Scientists like Full occupy a unique space in the academic community. They are renaissance researchers whose work falls somewhere within the intersection of biology, physics, mathematics, computer science, engineering, and robotics. Their work is also informed by a sprinkling of materials science, neuroscience, design, and even philosophy. That’s because building a robot requires solving myriad problems. Researchers in different fields bring different perspectives, in part because each is seeking to answer a different question. The discoveries that emerge from these collaborations are more than any single field could ever manage on its own. Ultimately, everyone wins.
As biologists like Full have lifted the veil from one biological enigma after another, they have been welcomed into robotics, a field traditionally stocked with engineers and physicists. Now, it’s practically de rigueur for a new robotics project to look to nature as a muse.
But those researchers aren’t simply trying to build an exact machine replica. Instead, they wrestle with nature, stripping it down to its most functional elements, and then improve upon it. Biomimicry—what some refer to as “nature worship”—is frowned upon by this community. If biomimicry was truly an effective strategy, researchers are fond of saying, planes would look like giant birds with flapping wings and submarines would have flopping fish tails.
“We’re careful not to practice bimimicry since it could lead us to some strange design that’s irrelevant or worse,” says Daniel Koditschek, a professor of electrical and systems engineering at the University of Pennsylvania. “It’s extremely easy to go wrong.”
Biological Basics
Few bioinspired robots are as famous as Stickybot. You may not know it by name, but you might recognize the whirring gate it uses to scale vertical glass walls. Stickybot is a robot gecko.
Geckos have dazzled scientists with their ability to scamper up walls, zip across ceilings, and perch on slick window panes. If you manage to catch one of these nimble lizards and take a closer look at its toes, you’ll see a plethora of thin ridges, almost like a tightly packed file drawer. Take an even closer look—at about 700 times magnification—and the ridges blossom into a thick rug of forest-like tubes. Even closer, at about 30,000 times, and you’ll notice that each of these tubes are covered with 100 to 1,000 nanoscale split ends. There are around 2 billion of these microscopic tendrils on every gecko.
For years, scientists were convinced that these tiny structures were responsible for the gecko’s gravity-defying acrobatics. But they had no idea how, exactly, until Full and his lab solved the biological riddle. They tested hypothesis after hypothesis, from velcro-like behavior to suction and glue. One by one, Full and his colleagues shot them down until they discovered that those hairy tips used the van der Waals force, which is the subtle attraction and repulsion between molecules. Following that breakthrough, mechanical engineer Mark Cutkosky and his colleagues at Stanford University created Stickybot. The robot’s footpads sport hairs that are much larger than the gecko’s 200 nanometer threads, but they work using the same principles.
Stickybot took the robotics world by storm, inspiring dozens of other labs to look at everything from kangaroos to cheetahs, moths, cockroaches, crabs, and flies. From those studies, researchers learned the basic principles of movement in the animal kingdom. All legged animals, for example, run by using a spring-loaded inverted pendulum pattern, similar to that of a pogo stick or bouncing ball. And lizard tails and ant feet, they found, both work as tripods to keep their owners stable on walls or in steep tunnels.
For now, Stickybot largely remains a laboratory curiosity.
Cutkosky’s robot was a success, but its utility was limited. It proved that researchers could build a robot that could climb like a gecko, but since it lacked a flexible spine, it could only manage to climb flat and gently curved walls. Also, it didn’t work well on rough or dirty surfaces. In other ways, its design hewed perhaps a little too closely to that of its real-world muse—the early generation Stickybot I mimicked the way geckos flex their toes to peel away from the wall, a burdensome feature that researchers have abandoned with Stickybot III.
For now, Stickybot largely remains a laboratory curiosity, though researchers are using it to develop the next generation of wall-crawlers. Now that they’ve built an approximation of the real thing, they can tailor the next versions—some of which will not resemble their living lizard inspirations. This next generation will be able to march up walls with loads that are far heavier than any gecko could manage. They’ll also be tailored for specific tasks, from snatching space debris out of orbit to forming landing perches for unmanned aerial vehicles.
“You’re peeling away all the layers of biological details that are not important for locomotion, only getting at the essence,” explains Young-Hui Chang, director of the comparative neuromechanics laboratory at Georgia Institute of Technology. “Engineering can surpass biology, but the key is to search wisely, not just imitate for the sake of imitating.”
Terrain Travails
Back at Carnegie Mellon, Choset was having no problem sending his snakes slithering—or springing—across a tiled floor. But he knew that if his robot was to succeed in the real world, it would have to contend with all sorts of terrain. For example, a snake robot sent through a collapsed building on a search-and-rescue mission may need to navigate tight pipes, uneven rubble, steep inclines, and even water. Many real-world snakes could do that if they needed to, improvising where they had to, but a robot snake needs its movements to be preprogrammed. “Snakes are really the masters of taking advantage of surface topography,” says David Hu, a mechanical engineer at the Georgia Institute of Technology. “But people still don’t understand why snakes can do all the things they can do.”
To bring his robots up to par with real-world snakes, Choset has distilled snake locomotion into a set of equations. By tinkering with the models’ parameters, he can emulate different snake gaits. Until recently, Choset’s snakes had mastered three of the four main gaits that real-world snakes use to slither through the environment. (They could also perform several more gaits that muscle and bone-bound animals cannot.) He has developed various models, including one that went inside a patient’s body to assist in mapping the outside of her heart. Another of Choset’s snake robots specializes in manufacturing and is being commercialized by a major company. Still others have explored archeological sites in Egypt, and he’s putting the final touches on another that can dive 200 feet down in the ocean.
Yet for all of those technological feats, Choset’s prize snake robot, the Modsnake, could not easily scale a sandy slope. That elusive fourth gait—sidewinding—still eluded him.
To crack the puzzle, Choset drew upon a friendly rivalry with Dan Goldman, a physicist at the Georgia Institute of Technology. Goldman’s diverse background—a childhood spent collecting snakes and lizards, a graduate education in chaos theory, nonlinear dynamics, and granular media, and a postdoctoral stint in Full’s lab—provided him with a unique mix of tools. Back when he began working with Full in 2003, Goldman found that researchers knew very little about the way animals move through soil and sand, so he took that on as a speciality. He has since worked with a variety of sand-loving creatures, including sandfish lizards, ants, Zebra-tailed lizards, shovel-nose snakes, baby sea turtles, and solpugids, an order of arachnids.
“I realized there’s this entire universe out there that people know very little about, and my interest and skill sets happened to be useful for that,” Goldman says. “Climbing up a sandy hill sounds like a pretty boring task, but once you understand the principles, you can implement it in other ways and into other systems.”
When Goldman heard that Choset’s lab was struggling with subtleties of the sidewinder’s movements, he, Hu, and two of their students decided to take a stab at this latest sand-related problem. “Their snake robot was loosely based on a snake in the sense that it was long and skinny, but that was it,” Goldman says. “Until our study, they had no insight from biology on how to make it better.”
So Goldman and his collaborators approached the problem from a different perspective. They convinced the Atlanta Zoo to build a special facility for housing venomous sidewinder snakes from the Arizona desert, bringing 300 pounds of sand from that same desert along with the animals. Then they pored over hours of footage of snakes crawling up various sandy inclines to determine the kinematics and physics that underlie sand-body interactions.
“This is an example of robots, biology, and physics coming together.”
Soon, they picked up on a “cool trick” that sidewinders use to mitigate slips when climbing granular surfaces: they flatten themselves out like an ellipse, increasing their contact with the ground. With the added friction, they can wiggle their way up steep slopes, effectively making the shifty sand behave more like a solid.
Choset seized upon this new data. He altered the parameters in his snake models, tweaking the Modsnake’s gait to approximate the key flattening motion. Rather than directly flattening, the robot simply changes its degree of contact with the sandy surface. “We were able to adjust parameters in our gait model to copy the behavior that Dan observed,” Choset says. “This is an example of robots, biology, and physics coming together to better understand the animal, as well as make the robot work better.”
Without these sorts of interdisciplinary projects, “computer scientists end up with very beautiful factory robots that can move over hard floors, but those that can exist in nature are very limited,” Goldman says. “Studying organisms in their natural environment is a critical feature for creating the next generation of high-performing robots.”
The Next Generation
Ten years from now, Choset and several other researchers I spoke with predict a proliferation of bioinspired robots that will do everything from slink through the rubble of collapsed buildings searching for survivors, rove the planet’s deserts collecting data on climate change, buzz over war zones collecting intelligence, and labor in the homes of the elderly who can’t live alone. They might even make it to Mars, where engineers won’t have to worry about their billion-dollar rovers getting stuck in a crater.
As Choset’s growing repertoire of robot snakes demonstrate, some of these creations are coming along quickly. With an additional $5 million investment, he says, he could have a robot ready to inspect nuclear facilities in about two years.
Despite the field’s furious pace, there’s still a long way to go. While robot designers can free themselves from limitations imposed by nature, they must also deal with the shortcomings of machinery. Take sensors, for example. “The difference between real animals and things that seem life-like is that animals can really sense the environment and respond,” Hu says. “The smallest ant may have 1 million times more sensors than the most complicated robot.” Energy, too, is a limitation. Batteries can only hold so much power, but more vexing is that robots tend to move less efficiently compared with animals. “We’re so far behind nature,” Choset says. “It’s humbling and sad, but it’s a good goal for us to strive for.”
“This is just about the most exciting field of science there is.”
As more universities create melting pots of disciplines, additional minds and collaborations will likely accelerate the process of discovery. “I tell my students, this is just about the most exciting field of science there is,” Koditschek says. Many of them are now seeking training in biology, engineering, or physics, fields that were once largely separated from one another. Biologists are now finding jobs in physics departments and vice versa. Some universities are formally blurring the lines, creating interdisciplinary graduate programs that offer biologists the quantitative training they need to understand engineering while giving engineers the foundation in evolutionary science to help them understand why animals look and behave the way they do.
Taken together, they amount to a sea change in the way we envision, develop, and deploy machines. “I think it’s going to be a crazy time in the next 20 to 30 years,” Goldman says. “Once we have better models, then we can really take our gloves off and design.”
Photos courtesy Howie Choset, furryscalyman/Flickr (CC-BY-SA) , Stanford University, and eiswuerfel Flickr (CC-BY-NC)