The Legacy of E = mc2
What hasn't Einstein's iconic equation touched in our world?
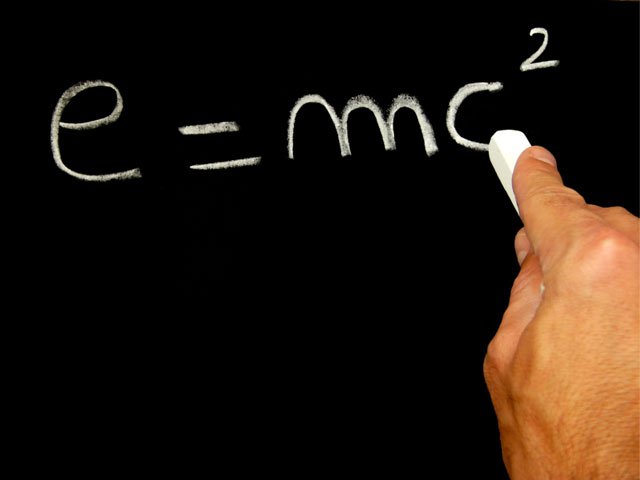
The equation explained
First, though, a capsule explanation of "energy equals mass times the speed of light squared" might be helpful. On the most basic level, the equation says that energy and mass (matter) are interchangeable; they are different forms of the same thing. Under the right conditions, energy can become mass, and vice versa. We humans don't see them that way—how can a beam of light and a walnut, say, be different forms of the same thing?—but Nature does.
So why would you have to multiply the mass of that walnut by the speed of light to determine how much energy is bound up inside it? The reason is that whenever you convert part of a walnut or any other piece of matter to pure energy, the resulting energy is by definition moving at the speed of light. Pure energy is electromagnetic radiation—whether light or X-rays or whatever—and electromagnetic radiation travels at a constant speed of roughly 670,000,000 miles per hour.
Why, then, do you have to square the speed of light? It has to do with the nature of energy. When something is moving four times as fast as something else, it doesn't have four times the energy but rather 16 times the energy—in other words, that figure is squared. So the speed of light squared is the conversion factor that decides just how much energy lies captured within a walnut or any other chunk of matter. And because the speed of light squared is a huge number—448,900,000,000,000,000 in units of mph—the amount of energy bound up into even the smallest mass is truly mind-boggling (see The Power of Tiny Things.)
Of course, intuitively understanding that energy and matter are essentially one, as well as why and how so much energy can be wrapped up in even minute bits of matter, is another thing. And E = mc2, which focuses on matter at rest, is a simplified version of a more elaborate equation that Einstein devised, which also takes into account matter in motion (more on that in a moment). But I hope that you, like I, now have a basic comprehension with which to appreciate the equation's prodigious influence. Perhaps the equation's most far-reaching legacy is that it provides the key to understanding the most basic natural processes of the universe, from microscopic radioactivity to the Big Bang itself. Radioactivity is E = mc2 in miniature. Einstein himself suspected this even as he devised the equation. In the 1905 paper in which he introduced E = mc2 to the world, he suggested that it might be possible to test his theory about the equation using radium, an ounce of which, as Marie Curie had discovered not long before, continuously emits 4,000 calories of heat per hour. Einstein believed that radium was constantly converting part of its mass to energy exactly as his equation specified. He was eventually proved right. Today we know radioactivity to be a property possessed by some unstable elements, such as uranium, or isotopes, such as carbon 14, of spontaneously emitting energetic particles as their atomic nuclei disintegrate. They are metamorphosing mass into energy in direct accordance with Einstein's equation. We take advantage of that realization today in many technologies. PET scans and similar diagnostics used in hospitals, for example, make use of E = mc2. "Whenever you use a radioactive substance to illuminate processes in the human body, you're paying direct homage to Einstein's insight," says Sylvester James Gates, a physicist at the University of Maryland. Many everyday devices, from smoke detectors to exit signs, also host an ongoing, invisible fireworks of E = mc2 transformations. Radiocarbon dating, which archeologists use to date ancient material, is yet another application of the formula. "The decay products that we see in carbon dating—that energy is directly obtained from the missing mass that you see in E = mc2," Gates says.Heavenly applications
Space technologies owe much to the equation. Unceasing E = mc2 disintegrations from radioactive elements such as plutonium provide everything from power for telecommunications satellites to the heat needed to keep the Mars rovers functioning during the frigid martian winter. Space travel in the distant future may also rely on such radiation-derived power. Photons streaming out from the sun and other stars hold energy that in the vacuum of space can theoretically be harnessed to propel a spaceship. "In the far future," says David Hogg, a cosmologist at New York University, "if you imagine that we're sailing to distant stars with spaceships that are driven by radiation pressure—if that ever happens, that will be a really big legacy of Einstein's kinematics.""One of its legacies is very sociological—it just captures the imagination of everyone."
(If you must know, it's E2 = m2c4 + p2c2, where p equals momentum.) "His bigger equation plays an enormous part in our understanding of how light works, and how energy and light can be transferred and transformed from one place to another, and that sort of thing," Gates says. "So if you consider the larger context, the part of the equation that's not in the public eye, it has an even larger legacy in science."
One application that draws on this larger equation, Gates says, is the giant neutrino detector now being built in Antarctica. Sunk deep in the ice, it will detect the eerie blue light, known as Cherenkov radiation, that is given off by neutrinos. Neutrinos are subatomic particles so lacking in mass that they pass straight through the Earth unscathed. Studying their light helps cosmologists better understand these mysterious particles and their distant sources, which may include black holes. Thus, says Gates, "as part of the equation's legacy, we'll be using the ice of Antarctica to look at neutrinos and other objects coming from outer space. And without knowing the relationship between the energy, momentum, and mass, that would be inconceivable to do. In fact, it was the use of this equation that led to the realization that neutrinos must exist."
A nuclear world
Einstein's equation also perfectly describes what's happening when we produce nuclear energy. As Arlin Crotts, a professor of astronomy at Columbia University, puts it, "our entire understanding of nuclear processes would be sort of lost without it." Fission reactors in nuclear power plants generate electricity by unlocking the energy tied up in fissionable materials. Fusion also furnishes energy from mass just as the equation posits. When two hydrogen atoms fuse to form a helium atom, the mass of the resulting helium is less than the two hydrogens, with the missing mass manifesting itself as fusion energy. Nuclear weapons, too, operate on the principle defined by the equation. Indeed, the mushroom cloud of an atomic bomb explosion is E = mc2 made visible. The equation spawned a whole new branch of science—high-energy particle physics. Labs that work in this field thrive on E = mc2 conversions. In fact, proper design of particle accelerators, as well as analysis of the high-speed collisions within them, would be impossible without a thorough comprehension of the equation. Within accelerators, colliding particles are constantly vanishing, leaving only energy, and dollops of energy are constantly transmuting into newly fashioned particles. "Our species has repeatedly used an understanding of the equation to convert E into new forms of m that had never previously been seen," Gates says. "One of the outposts of science for the next century may well be whether the E includes super-E, and m includes super-m—new forms of energy and matter called 'super-partners.'" A grasp of the equivalence of mass and energy also comes in handy when studying antimatter. When a particle meets its antiparticle, they annihilate each other, leaving only a pulse of energy; by the same token, a high-energy photon can suddenly become a particle-antiparticle pair. Altogether, says Hogg, "E = mc2 has been very important in diagnosing and understanding properties of antimatter." Einstein's formula also accounts for the heat in our planet's crust, which is kept warm by a steady barrage of E = mc2 conversions occurring within unstable radioactive elements such as uranium and thorium. "When they decay, some of the mass is lost and a little energy is created, and that keeps the crust warm," says John Rigden, a physicist at Washington University in St. Louis and author of Einstein 1905: The Standard of Greatness (Harvard, 2005). "So the temperature of the outer Earth, the crustal matter, is directly related to E = mc2."A cosmological constant
A similar process happens far beyond Earth, inside stars. The warmth we feel from the sun, for example, is the result of the energy generated as hydrogen deep within our star continuously fuses to form helium. And stars don't stop there. When they exhaust their hydrogen, they begin to burn new fuels and create new elements, which are spewed out into the universe when the stars eventually explode, as burnt-out stars are wont to do. "The carbon, oxygen, nitrogen, and hydrogen that make up living organisms were baked in the innards of a star," Rigden says. "In terms of what goes on in stars, we owe our existence to E = mc2." Einstein's equation even tells of what transpires at black holes, which can contain the mass of millions of stars. Here, E = mc2 is taking place on an astronomical—and highly efficient—scale. "In a nuclear process, you convert something like one part in 1,000 of your rest mass into energy, whereas if you fall into a black hole, you can convert something like 20, 30, 40 percent," Hogg says. "So from the point of view of the energetics of the universe, these black holes are important, because they are big converters of rest mass into energy.""That's one of the legacies—that we've learned the power of human creativity in the sciences."