 |
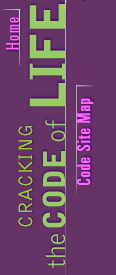 |
|
Dr. Eric Lander
|
Meet the Decoders
Dr. Eric Lander
Krulwich: Let me see, let me just start with the gene itself. First of all,
we're all familiar with this thing. This shape is very familiar.
Lander: Double helix, yes.
Krulwich: Double helix. First of all, this is my version of a DNA molecule, but
I'm just curious how small is it in real life? Like the distance--is this by
the way, what it looks like?
Lander: Well, give or take, a cartoon version. Yes. That's right.
Krulwich: This is made of what, these sort of walls?
Lander: This chain here that runs along the outside is made of sugar molecules
and negatively charged phosphate molecules. Then it goes:
sugar/phosphate/sugar/phosphate/sugar/phosphate, running all along here, boring
and utterly repetitive.
Krulwich: So if you lick it, it would be a little bit sweet this part?
Lander: Well, no. It wouldn't because they're all a patch. You see, it only
tastes sweet if it comes off and activates your taste receptors. So these long
chains are not very good.
Krulwich: Okay.
Lander: I mean wood is also made out of these sugars and things, and it doesn't
taste very sweet, because it's all sown up.
Krulwich: Okay.
Lander: But if you broke it all up, there is a little sugar in there.
Krulwich: The main thing about this is the ladder, the steps of this
ladder.
Lander: So the news is along these steps, right? The outside is the same all
along; the inside sticking out of these sugars comes one of four different
things, one of four bases that we call A, T, C, or G. They correspond to
different funny molecular shapes with little rings and things.
Krulwich: And when you say A, T, C -- I'm just going to set this thing up
here.
Lander: Okay. So let's say that this red guy here is an A and that's a
T.
Krulwich: Why do you call them A and T?
Lander: Oh, it's adenine and thymine.
Krulwich: So those are their initials?
Lander: But it's a fast-moving field. You can't say adenine all the time and
thymine and cytosine all the time and guanine all the time, so you say, A, T,
C, and G.
Krulwich: So there are in almost every cell in your body, if you look deep
enough, you will find this chain here?
Lander: Oh, yes. Stuck to the nucleus of your cell there is I guess 23 pairs of
these chains called chromosomes.
Krulwich: Right.
Lander: Each chromosome is a very long chain of about anywhere 100 million
rungs of the ladder.
Krulwich: In every cell?
Lander: Every cell.
Krulwich: Over and over. How many of these steps do we have in a typical cell
in me?
Lander: Well, you've got six billion, of which three billion came from your mom
and three came from your dad. Those two copies are pretty similar, so every
cell has a genome of three billion, and it actually has about--it has two
copies of it; one from mom and one from dad.
Krulwich: Let's just think for a moment. Let's just look at the whole
landscape. The human being has 3.1 billion of these letters, not all of them
are genes, in fact only 1 percent of them are genes. So, where are they? Is
there any pattern?
Lander: The genome is very lumpy.
Krulwich: Very?
Lander: Very lumpy, very uneven. You might think, if we have 30,000 genes,
they're kind of distributed uniformly across the chromosomes. Not so. They're
distributed like people are distributed in America: they're all bunched up in
some places, and then you have vast plains that don't have a lot of people in
them. It's like that with the genes. There are really gene dense regions, that
might have 15 times the density of genes, sort of New York City over here. And
there are other regions that might go for two million letters and there's not a
gene to be found in there.
Krulwich: So there's this whole conversation going on that we didn't know
about, like, ten years ago.
Lander: The genome is, it's a fossil record; the genome is a landscape; the
genome is a whole geography of distributions. The thing about the human genome
that most surprised me was how many amazing stories there were in it. That you
might think the genome's just a boring string of letters, like reading the ones
and zeros on your hard disk. The genome is a storybook that's been edited for a
couple of billion years, and you could take it to bed, like A Thousand and
One Arabian Nights, and read a different story, in the genome, every
night.
Krulwich: If I could read each of the individual letters, I might find the
picture of what?
Lander: Well, of your children. This is what you pass to your children. You
know, people have known for 2,000 years that your kids look a lot like you.
Well, it's because you must pass them something, some instructions that give
them the eyes they have and the hair color they have and the nose shape they
do. And the only way you pass it to them is in these sentences. That's it! It
is hereditary.
Krulwich: So if I decode the two parts of each of these ladders, I would know
many things about my children, things to be.
Lander: Well, in principle you would, the same way that if you read all the
ones and zeroes on your hard disk, you would in principle know the Mozart
symphony or the Shakespeare play that you put on your hard disk. Now, whether
you could actually take the ones and zeroes and sing the Mozart symphony from
that, is another question. So getting the letters out is one thing. Extracting
their meaning is another. The Human Genome Project is about sitting there and
getting the letters out. The next century is about extracting all of the
meaning out of the text, but it's a pretty good text. It's worth the effort.
Krulwich: Getting the letters out has been described as finding the blueprint
of a human being, finding a manual for a human being, finding the code of a
human being. What's your metaphor?
Lander: Oh, golly, gee, I mean -- I think this is very much like in chemistry,
the way the chemists describe all of matter in terms of elements that they put
into a periodic table.
Krulwich: Right.
Lander: I think this is kind of biology's periodic table. Everything that gets
made in your body, whether it's, you know, carotene in your hair or collagen in
your skin or hemoglobin in your blood, is specified by an instruction here.
This is basically a parts list. Blueprints and all these fancy -- it's just a
parts list. It's a parts list with a lot of parts.
If you take an airplane, a Boeing 777, I think it has like 100,000 parts. If I
gave you a parts list for the Boeing 777, in one sense you'd know a lot. You'd
know 100,000 components that have got to be there, screws and wires and the
rudders and things like that. On the other hand, I bet you wouldn't know how to
put it together. And I bet you wouldn't know why it flies.
Well, we're in the same boat. We now have a parts list. That's what the Human
Genome Project is about is getting a parts list. If you want to understand the
plane, you have to have the parts list, but that's not enough to understand why
it flies. Of course, you'd be crazy not to start with the parts list. So we
figured that for the next century of medical work, we'd better get the parts
list and so everybody rolled up their sleeves and decided we could work
together and get a parts list.
Krulwich: Before we go to how we do that, let me just give you what I guess is
one advantage. If I discover that some human being is sick, and I suspect that
the sickness comes from some genetic trait, I guess I could ask and ask and ask
this molecule, "What's wrong with my kid? What's wrong with my kid? What's
wrong with my kid?" But the fact that it's a molecule with a beginning and an
end means that whatever the mistake is it's somewhere in this molecule.
Lander: That's what's so important about this being like a periodic table. When
the chemists try to go figure something out, they know there's only 100
elements or so, and whatever you're trying to explain about matter, you've got
to explain in terms of those 100 elements -- oxygen, hydrogen, etc.
What's now happening in biology is we know that whatever you want to explain
about heredity it's got to be in the three billion letters specifying maybe
30,000 genes or so. In the past you could always say, "Well, the cause is
something we haven't discovered yet." Not anymore. There isn't a something we
haven't discovered yet in genome form, or there won't be very soon, and so it's
a sea change in science to know that you're explaining cancer or you're
explaining brain degeneration that's inherited, whatever you're explaining, you
only have 30,000 explanations to use and maybe their combinations.
Everybody looks for the genome and thinks, "Ah! We're going to cure
everything." No. It doesn't mean we're going to cure everything. It means for
the first time we stand a fighting chance of explaining the causes of things
and getting understanding about how it works. And then sometimes we'll be able
to cure it by the understanding. It's just that up to now we've been trying to
do it in utter ignorance about most of the parts.
Krulwich: Most people think that this project has to do with getting sick, that
is, you're going to discover the gene that causes blindness and the gene that
causes deafness and the genes that cause cancer and the genes that cause these
terrible things. But aren't we also trying to figure out the gene that make you
just a bit different from me, so we could discover the chemistry of difference?
Or is that not the issue?
Lander: We want to understand what makes a human being tick. Sometimes we study
differences between people, and the best way to find out what's wrong common to
all of us. By figuring out why I'm taller than someone who is shorter, we can
figure out the common mechanism that controls bone growth, for example. And
that mechanism is useful to everybody, so in some sense all this focus on
difference is not really about classifying people by their differences, it's
that differences are our best way in to the biological mechanisms that are the
same in all of us.
We can only study those mechanisms when we see a difference in them, because
that's our clue to know that the mechanism is there. If we were all exactly the
same height, we wouldn't think much about the mechanism that controls height,
but because we see a lot of difference in height, we're clued in to the fact
that there are mechanisms at work that make some of us taller than others, and
we can study those.
Krulwich: How will we know the difference that genes make and the difference
that environment makes? For example, if we're looking for cancer-causing
agents, and we go to Finland, we might say after rigorous study of the people
of Finland that everybody who gets cancer there seems to have a problem, here,
here, and here. But then when we go to Tanzania, we may discover that everybody
who gets cancer in Tanzania has a problem here the same, but now here and here.
Then what have we learned?
Lander: Well, the first thing to say is that the differences around the world
are much less than you think, even though we're six billion people scattered
around the whole world. In point of fact, the entire human species traces back
a mere 5,000 generations ago, to a small population in Africa. All of us
descend from a population of 10- or 20- or 30,000 people in Africa about
100,000 years ago. And it means that, because 5,000 generations is such a short
time from the point of view of genetic evolution, the variance present in
Africa and the variance present in Asia and the variance present in northern
Europe are largely the same. There's not that much variation.
Krulwich: How close are we? I know that we are very close to chimpanzees for
example.
Lander: Any two chimps in Africa are five times more different than any two
humans on this globe.
Krulwich: Any two chimps?
Lander: Any two chimps in Africa have five times more genetic variation than
two random humans on this globe. You think the chimps all look the same;
believe me, they think we all look the same.
Krulwich: And on paper, anyway, we are more of the same to each other?
Lander: We are much more similar to each other than chimps in Africa
are.
Krulwich: Because we are such a young species?
Lander: We are a small species that has grown large in the blink of an eye. We
tend to forget that; we like to focus on our differences, but our differences
are a pretty trivial portion of our genome. And the distribution of those
differences across the globe is actually much flatter than we think about, much
more uniform. If we take any village anywhere in the world, it has roughly 80
percent of all the genetic variation found anywhere in the world, just within
that village. We just like to have wars and things about how different we all
look, but compared to most species, we're not very different.
Krulwich: If we go down that list of 3.1 billion chemical constituents.
Lander: Yup.
Krulwich: Do all of them manufacture something that makes us?
Lander: No. No. Actually most of the DNA letters in your genome don't
manufacture any proteins that do anything in your body. Indeed, only a few
percent are probably needed to manufacture all the proteins in your
body.
Krulwich: Why do you mention proteins?
Lander: Genes contain instructions for making things, and almost always the
things that they contain the instructions for are proteins like carotene in
your hair or collagen in your skin or hemoglobin in your blood.
Krulwich: Huh! So genes create proteins and proteins build us.
Lander: Proteins do the work. Genes are the instruction sets for those
proteins. Actually only a few percent of all of your DNA is devoted to writing
down the instructions for those proteins.
Krulwich: Say if five percent makes the proteins, what does the other 95
percent do?
Lander: Well, at least 50 percent of it consists of selfish DNA
elements.
Krulwich: Of what?
Lander: Selfish DNA elements, that is to say chunks of DNA that know how to
reproduce themselves. And they just live in your chromosomes, so you think it's
your genome, but, in fact, more than half of your genome consists of elements
that know how to copy and move themselves around or fossils of such
elements.
Krulwich: Are they my predecessors up the genealogical tree?
Lander: No. No.
Krulwich: Are they a snake that I formally was?
Lander: Not at all. They couldn't care less about the snake or anything like
that. They've been around for about one and a half billion years, and they're
little DNA segments. There's one that's about 6,000 letters long. It knows how
to copy itself into RNA and move itself to someplace else in your DNA, and when
it gets there, it knows how to copy itself and move itself someplace else. It
doesn't do anything useful for you, and, thank you, it isn't interested in
doing anything useful for you.
Krulwich: Is this some billiard ball that I've got wandering around inside my
genome?
Lander: Well, it's something like that, yes. You've got these things hopping
around your genome that get called "transposable elements," and they look out
for themselves. They're like little parasites in your genome.
Krulwich: Okay. So I've got letters that do stuff that makes me.
Lander: Right.
Krulwich: I've got wandering around stuff that doesn't do anything. It just
seems to have the habit of living in me.
Lander: It does a lot for itself. It largely thinks your genes are just there
to help propagate it.
Krulwich: So I've got five percent that's making me, a lot of stuff that's
jumping around and then trash or junk?
Lander: It's junk. You keep it around, because it turns out that occasionally
the junk in your genome turns out to be very handy. Every once in a while a
piece of junk that lands in your genome serves a useful purpose and gets used
by the body. A great example is your immune system. Your immune system does a
very cute trick where it can rearrange pieces of DNA to make antibodies to
recognize anything. You know where it learns how to do that?
Krulwich: Where?
Lander: It's a piece of junk that landed in the genome about 400 million years
ago that carried with it an enzyme for hopping around. Your body took over that
enzyme and used it to rearrange genes to make antibodies. So every once in a
while we get quite a nice gift from this junk.
Krulwich: So you make no apologies for spending all those long machine hours
writing down the names and letters of all that stuff.
Lander: Indeed, many people argued in the 1980's that we should just spend the
money, sequencing the important stuff. The problem is the important stuff is
scattered about in the sequence. We could have spent 20 times as much money,
just finding the important stuff and sequencing it, as sequencing
everything.
It would be like, you know, going through a library and, you know, reading the
books or something and trying to -- "I'm just going to read the interesting
sentences." Well, it's really tough when you've got a novel to just read the
interesting sentences or the interesting paragraphs without reading the novel.
Now somebody will invent a very clever way to do that, but nobody has yet, and
so the most efficient way was to sit there and read the whole thing. It turned
out to be not that expensive, and every one of those letters helps us
understand human biology. We can actually learn a lot from the junk. You want a
great example?
Krulwich: Yeah.
Lander: The gene that causes Duchenne muscular dystrophy when it's mutated,
that gene is spread out over two million letters of DNA, of which only 16,000
matter.
Krulwich: Wow! So the useful part is scattered in the junky part?
Lander: The cell copies and copies and copies two million letters, and then it
splices together, throwing out almost all two million letters to save only
16,000 letters to make that protein.
Krulwich: But how do you know of all these rungs which is the stuff that's
doing stuff for you?
Lander: That's what we're engaged in right now. That's called interpreting the
sequence, annotating the sequence. You've got all the letters. It actually
doesn't come color-coded; it doesn't come with little special marks saying
"This is important." You have to sit there with all the A's, T's, C's and G's
and figure out which bits matter. And so we have elaborate computer programs to
try to figure out which fits have the potential to code for a protein in your
body.
Krulwich: I get the sense that everybody is getting out of the gene business
and suddenly going into this new business I hear about called the protein
business. There's even a new name, instead of the genome, I'm hearing this
other name, which I don't--
Lander: The proteome.
Krulwich: The proteome, what is that?
Lander: Well, the genome is the collection of all your genes and DNA, the
proteome is the collection of all of your proteins. See what's happening is
we're realizing -- I think we always realized that if we wanted to understand
life, we had to start systematically at the bottom and get all the building
blocks. The first building blocks are the DNA letters, from them we can infer
the genes. From the genes, we can infer the protein products that they make to
do all the work of your cell.
Then we've got to understand what each of those proteins does, what its shape
is. How they interact with each other, and how they make kind of circuits and
connections with each other. So in some sense, this is just the beginning of a
very comprehensive systematic program to understand all the components and how
they all connect with each other.
Krulwich: How many proteins do we have?
Lander: Well, it's very interesting. One gene can make multiple proteins. It
turns out that the gene makes a message, but the message can be spliced up in
different ways, and so a gene might make three proteins or four proteins, and
then that protein can get modified. There could be other proteins that stick
some phosphate group on it or two phosphate groups.
And, in fact, all of these modifications to the proteins could make them
function differently. So, while you might only have say, 30,000 genes, you
could have 100,000 distinct proteins, and when you're done putting all the
different modifications on them, there might be a million of them. It's scary
talk.
Krulwich: The proteome project could then last a lot longer than the genome
project?
Lander: Oh, no. I doubt it, because, I mean, the difference between 30,000 and
100,000 is only a factor of three. The great thing about this explosive period
of science is that these big numbers, they sound scary, and then within five
years some bright student figures out a way to say, "Oh, yeah, well, what's a
factor of five, and what's a factor of 10 here and there?" It wasn't very long
ago that sequencing a couple hundred letters of DNA was enough to get a whole
Ph.D. thesis.
Krulwich: Now a robot does it?
Lander: A robot does an awful lot more than that in the course of a day.
There's just this incredibly explosive learning curve about how to work with
this material.
Krulwich: Before I leave the subject of proteins, one last question.
Lander: Yeah.
Krulwich: When I think about proteins, if I'm trying to think strategically --
I think if I get sick that may mean that my proteins are causing me harm,
because the bad gene created a bad protein?
Lander: Yup.
Krulwich: So are pharmaceutical companies now going to be trying to figure out
how to maybe not get down and rearrange the genes, but maybe just deal with the
protein?
Lander: Most drugs on the market today affect the protein, not the gene. The
gene is the instruction for making this component. The drug you take, the pill
you pop, usually interacts with the protein, to slow down its function, speed
up its function. That's what's really going on. Very few drugs interact with
genes.
Krulwich: So if I had a horrible genetic disease, like Huntington's, maybe
someone will figure out a way to cure the protein part of my disease?
Lander: For Huntington's disease we know that the problem is the gene is
defective in a way that it makes a protein that is a big long extra chunk of it
that's very sticky, and it sticks to other stuff and gums up the works. The
Holy Grail for Huntington's disease is to make a drug that would block this
sticky protein from interacting with other things.
Krulwich: How about cystic fibrosis?
Lander: For cystic fibrosis, it's a little trickier. There you have a protein
that can't perform its job. It can't transport chloride across itself. You've
got to make a drug there that somehow would restore its function. That's not
very easy. When you have a broken part, it's hard to add a gene that will
restore its function. So there people are talking about somehow spraying a
virus into your lungs that will carry with it a new good copy of the cystic
fibrosis gene, for example. Those are the kind of ideas people have.
Krulwich: And, last, I get the sense that the genome project was largely a way
of discovering a long chain of things? When I think about the proteins and the
proteome project, for some reason I think about origami. I don't know why, but
when I read things, I hear that you're folding and unfolding and bending and
twisting. What is that about?
Lander: The genome project was a piece of cake compared to most other things,
because genetic information is linear. It goes in a simple line up and down the
chromosome. Once you start talking about the three-dimensional shapes into
which protein changed and can fold, and how they can speak to each other in
many different ways to do things, or the ways in which cells can interact like
wiring up in your brain, you're not in a one-dimensional problem anymore.
You're not in Kansas anymore. You're way off.
Krulwich: You need geometry--
Lander: You need complicated geometry and diffusion. That's why it's not a
quick shot to just go from the genome to curing something. The work of the next
century will be to take the simple linear sequence and see if we can build
models that explain these complex three-dimensional interactions and the kind
of conceptual circuits of A tickles B, tickles C, tickles D at the molecular
level and figure out, you know, which proteins to goose to fix some
disease.
Krulwich: Here's one of the big astonishments, to me. We are related, more
closely related, apparently, to creatures, than I had ever imagined. You
too?
Lander: We are all incredibly related to creatures.
Krulwich: But a chimpanzee, that I could figure. A big ape, that I could
figure. Eighty to eighty-five per cent of the genes in a mouse are in
one.
Lander: More than that.
Krulwich: More than that.
Lander: More than that. I'd say, it's 98 percent of the genes in the mouse, you
can find clear matches in a human. There's not a lot of difference between you
and a mouse.
Krulwich: What does that mean exactly? If you took off the skin of a mouse,
went right in and looked at the cell, would you just see the same exact stuff,
in the same exact order that you see in me?
Lander: If you looked inside the body of a mouse, you see lungs, you see
hearts, you see pancreases.
Krulwich: But let's get cellular.
Lander: But wait a second. All of the genes that have to direct the arrangement
of those organs have to be the same. The only difference is, a few genes might
control some size. There might be a little variation of the exact shape of
things. But those are small compared to building all these complex structures.
Pretty much every structure we have a mouse has. And so most of the genes
you're going to need to build it are the same. It's like two kinds of
airplanes.
Krulwich: But we are so much bigger, smarter. We make music, they squeak. We
walk around, we go to school. There has, as far as I know, never been a mouse
school.
Lander: Yeah.
Krulwich: Therefore, how could they have as many genes as we do and yet we be
so much, excuse the expression, better.
Lander: Well, it's a somewhat self-centered view to think we're better, but
we're certainly different, right? I think the main take-home message from this
is that the differences in the human from many other organisms may actually be
small differences of degree that then have gotten amplified.
See, we do all sorts of things that a chimp doesn't do. And that's not because
we invented a lot more genes. It may be for something as simple as the little
genetic controls that cause the number of nerve endings made them double in the
human, for example. That could lead to lots more connections without lots more
genes. It might be just tweaking the dials a little bit ends up producing quite
qualitatively different behavior.
Krulwich: So you could look deep into the eyes of a great ape or a chimpanzee
and there is something vaguely familiar about that.
Lander: Vaguely familiar? It's virtual identical. To any other organism on this
Earth, they'd have a hard time telling you apart from the great ape.
Krulwich: And yet what's the difference between us may be simply that our genes
turned on some dial so we got ten times more brain cells than they got, or
something like that?
Lander: Or, probably more likely, that brain development slowed down in humans
so that more of it could be affected by environment and experience and
childhood. In many ways, it said that the human brain development is more like
a baby chimpanzee, a neonatal chimpanzee, and that we are therefore open to
more learning and more shaping by environment for much longer in our
development. That may be the secret to it, that we actually fossilize our
brains into the adult form much more slowly.
Krulwich: Which means that the difference between us and the big ape is not the
genes that we don't have together, it's... What is it, then?
Lander: It may be the controls on these genes. It may be simply how soon the
gene is turned up or off or what level it's turned on to. It might be two
letters of DNA that affect how actively the gene is transcribed in the cell.
Those are the sort of small variations on the theme that could make a huge
difference in how an organism turns out.
Krulwich: Let me get back to relationships. If we are almost identical to mice
and 80 percent of the genes in the cow are in us, and 61 percent of the genes
in a fruit fly are in us, and -- this is the one that gets me -- 50 percent of
the genes in a banana are in us?
Lander: How different are you from a banana?
Krulwich: I feel -- and I feel I can this with some authority -- very different
from a banana.
Lander: You may feel different from a banana--
Krulwich: I eat a banana, but I have never--
Lander: Look, you've got cells, you've got to make those cells divide. All the
machinery for replicating your DNA, all the machinery for controlling the cell
cycle, the cell surface, for making nutrients -- all that's the same in you and
a banana.
Krulwich: Wait. You honestly feel, when you came to this subject, a genetic
relationship to the banana that was this strong?
Lander: Take baker's yeast. Baker's yeast we're related to, one and a half
billion years ago. You can take genes out of a baker's yeast that controlled
the basic cell processes like cell division, and you can put them into a human
cell, and they function. And, in fact, they're the very genes that cause cancer
in us, because they affect cell division and they affect cell division in
yeast.
Krulwich: Wait. In other words, if you had a person who was sick in a
particular way -- let's say his cells weren't breaking down properly or
multiplying properly. You could take a healthy gene from a yeast, stick it in
the person, and make the human feel better, fix the human?
Lander: It would be unethical to do it that way, but we do the reverse all the
time. You can take the gene out of the human, stick it into the yeast, to make
it play the same role as its evolutionary relative, one and a half billion
years ago. And it works. Presumably, it works in the other direction, too,
although we don't do it on patients.
Krulwich: So you were saying, then, that you could take a yeast that wasn't
feeling too good and then go into my cell and take a piece of me, a healthy
chunk of me, and fix the yeast?
Lander: Well, if there was a defect in how the yeast's cell division occurred,
your gene would work just fine in the yeast, and people do that experiment all
the time. We can do gene therapy to cure a sick yeast using a human gene.
Presumably, it works the other way, too, although we don't do it, because it's
not ethical. But even after one and a half billion years of evolutionary
separation, the parts are were still interchangeable for lots of these
genes.
Krulwich: Now, does that mean--I just want to make sure if I understand this
right. Does that mean when you look through those things that all the C's and
the A's and the T's and the G's, are you seeing the exact same letter sequences
in the exact same alignment? When you look at the yeast and you look at the
person, is it C-C-C-A-T-T?
Lander: It's eerie. The gene sequence is almost identical. There are some
genes, like ubiquition, that's 97 percent identical between humans and yeast,
even after a billion years of evolution.
Krulwich: Well, with a name like that, it's got to be.
Lander: Well, yeah. But then there are other genes where, when you look at
other genes, you can still see, for example, that out of the protein sequence,
oh, 60 percent of the amino acids in the protein are exactly the same, and
maybe 20 percent more are at least similar chemically. And there's no doubt
it's the same object, even though it's varied some over one and a half billion
years.
Krulwich: Now, is this why you can go to a pig, a big fat pig, and get the pig
insulin out of the pig and give it to a human diabetic? I mean, are we talking
about the same thing?
Lander: Pig insulin, human insulin, it's doing the same thing: it's interacting
with the same receptor on the surface, an insulin receptor making the same kind
of molecular signal. It's the same thing. Now, there're slight variations in
pig insulin and human insulin that could lead to problems of immunological
cross-reaction, but that's usually about details, small details in the insulin.
The function of the pig insulin is virtually identical to the function of the
human insulin.
Krulwich: So when you say interchangeable parts, that's actually become a
business: People take a product from a pig and give it to a sick human, and the
sick human uses the product and gets better, feels better.
Lander: Although these days we do it by cloning techniques where we can
actually get a bacteria to produce the human product, and that's even safer
still. But you've got to understand that, deep down, the fundamental mechanisms
of life were worked out only once on this planet and have gotten reused in
every organism on the planet. Evolution doesn't go reinvent something when it
doesn't have to.
Krulwich: Well, is it a headline that we are more closely related to each-- By
the way, before I ask that, how about plants? Let's suppose I had like a
mustard seed, I don't know, a fern -- I'm trying to think as distant from
myself as I can. So I walk up to a fern and I say Hello. What part of the fern
has already got more or less pieces of me in it?
Lander: Oh, golly. Mostly what you share in common with a plant are the basic
genes that run a cell. Because while you look very different from a plant,
standing back, the closer and closer you get to a cell, the more you see bag
with stuff in it and a nucleus. And most of those basic functions are the same.
I suppose if you look at two different automobiles driving on the street, they
may look very different, but if you look under the hood the engines look
remarkably similar. And I think that's true for most cells, whether it's in
plants or animals -- that, under the hood, the engine is pretty similar.
Krulwich: And that seems to be a major discovery of this project, so
far.
Lander: Well, this has been a discovery, I think, that's been dawning on us
with genome sequencing over the last couple of years, and the human sequence
has made very clear that we are not separate and different in any way there. We
are very much partaking of that same bag of tricks that evolution's been using
to make organisms all over this planet.
Krulwich: Let me go to the next headline. Fruit flies, as much as you might
admire them, are small and foreign like creatures to moi. Yet I discover that
the number of genes in a fruit fly is only about half the number of genes in
me.
Lander: Yeah. That's really bothersome to many people, that we only have about
twice as many genes as a fruit fly. Because we really like to think of
ourselves as a lot more than twice as complex as a fruit fly.
Krulwich: Don't you?
Lander: I certainly like to think of myself that way. And so it raises two
questions: Are we really more complex? Well, I think so. I think we've got lots
more different tissue types, cell types, interactions in our body.
Krulwich: Well, let's let things speak for themselves. You show me the fruit
fly that can compose like Mozart, and then I'll obviously--
Lander: Show you the human that can fly, right?
Krulwich: All right.
Lander: We all have our talents, right?
Krulwich: Yes. Anyway, but you feel somewhat diminished, don't you, a little
bit, at least at first blush, by this?
Lander: Well, at first blush the reaction is to be a little insulted that you
only have twice as many genes as a fruit fly. But when you begin to think about
it, you realize that complexity may not just be in the number of genes. Twice
as many genes may translate into four times or eight times or 16 times as much
complexity. And we can see from the genome sequences -- this is really one of
the most exciting things from the genome sequences -- ways in which our own
genes are more complex.
Krulwich: So, the difference, then, the possible difference, between a fruit
fly and a human is that the number of different splices and restitchings in a
human is just higher?
Lander: It seems we've got twice as many. So one of the levels at which we're
more complex than a fly is that a typical gene might get spliced and diced up
into twice as many products as in a fruit fly. That's one level of
complexity.
Krulwich: So twice as many proteins are being produced by the same gene in a
human than in a fruit fly.
Lander: That's one level of complexity. But there's more to it. When we
actually look at the proteins in the human, proteins are made out of building
blocks that we call modules. Humans haven't invented a lot of new modules I've
got to say. Most of our modules are the same as in the fruit flies. But we put
them together in more combinations. We call those architectures. We have more
different architectures for our proteins than a fruit fly does. And I think
what that does is it lets our proteins interact in lots more ways. We can make
lots more complex tinker toys out of these parts, because we've combined those
modules in twice or three times as many combinations.
Krulwich: So starting with the same raw ingredients, the fruit fly goes... But
the human, by somehow or other being able to arrange all the parts in many
different ways, can produce melodies perhaps.
Lander: Yes, although we're not that good at hearing the melodies yet. One of
the exciting things about reading the genome sequence now is we're getting a
glimpse at that complexity of the parts and how it's a symphony rather than a
simple tune. But it's not that easy to just read the sheet music there and hear
the symphony that's coming out of it.
Krulwich: But is a metaphor okay? Should we think of the fruit fly somewhat as
a creature that's learning to play chopsticks, and think of the human as a
creature whose genes have learned to play Mozart?
Lander: Yeah. That's probably a bit extreme. I mean, it's pretty fancy making a
fruit fly, too. I'd say it's way past chopsticks, and we may not quite be
Mozart, but there's no doubt that we have more lines of music coming in
together, in a much more complex melody that's coming out of it.
Krulwich: And so the bottom line question is, What is it that makes a human
being human? Since if you're sitting next to a fruit fly and he seems to have
almost the same number of ingredients that you do, the difference, the thing
that makes us human, is?
Lander: Well, the one thing that distinguishes human beings from all other
organisms on this planet is they're the only organisms that worry about what
distinguishes them from all other organisms on this planet. Other than
that--
Krulwich: I was hoping for some more architectural response.
Lander: I understand, but every other attempt that people have to say why
humans are distinctive usually fails when you look really hard. But we're the
only ones that worry about that question.
Krulwich: But, if I hadn't forced you, you'd say it had something to do with
the human being's genes able to produce more proteins and more variation among
the proteins.
Lander: Now, be careful. This isn't what makes humans different. This is what
makes vertebrates different. Because whatever you're claiming for the human at
the level of its genomic complexity, you've got to claim for the mouse and the
dolphin. So this is not distinctively human. If you ask, "What's distinctively
human about the human genome, compared to, say, a chimpanzee?" We haven't a
clue. In fact, if I gave you the three billion letters of the chimp, and the
three billion letters of the human, and I didn't tell you which was which,
without cheating by peeking at the right answer, no scientist on Earth could
tell you which was human and which was chimp.
Krulwich: But why? Isn't there somewhere you look for "hair all over" or "big
face?"
Lander: Of course, somewhere it says, "Hair all over the body" in the chimp
genome. But we don't know how to read that. It's there, but we don't know how
to know that somewhere it said, turn off the hair on most of the skin of the
body. That's our level of ignorance about this.
This is what the next century is about. See, we have the text, for the first
time. It's incredibly exciting. We can see sentences and nouns and verbs all
over the place. We haven't got the plot from all that. We've just barely got
the text, we know bits of the language. We don't yet know, subtly, how -- it's
embarrassing. I give you a cat and a dog, the two genomes. It wouldn't be easy
to tell which is which. We can't tell.
Krulwich: Let me ask you about another important difference. Imagine, if you
will, a Sumo wrestler on the one hand, and one of those Sports
Illustrated bathing suit beauties on the other -- two humans of strikingly
different physique. What is the genetic difference between the fat guy and the
skinny girl?
Lander: The genetic difference between any two people, whether it's a Sumo
wrestler or a Sports Illustrated bathing suit model -- one-tenth of a
percent. Those two, and any two people on this planet, are 99.9 percent
identical at the DNA level. It's only one letter in 1,000 difference.
Krulwich: So that one letter in 1,000 makes him look this way and her look that
way?
Lander: Well, of course, him and her has a little to do with X and Y
chromosomes, so we've go to take that out of the equation. But the picture I
always like to show is Wilt Chamberlain, basketball player, and Willie
Shoemaker, jockey. Both men, 99.9 percent identical, but one of them is almost
twice as tall as the other. What's going on? Well, it tells us that, first, as
a species, we are very, very closely related, because any two humans being 99.9
percent identical means that we're much more closely related than any two
chimpanzees in Africa.
Krulwich: But if you look at an Icelander, that Icelander has such a different
physique than an African.
Lander: How many genes does it take to change a physique?
Krulwich: How many genes does it take to change a physique?
Lander: Well, we don't know, but we know there's not a lot of gene differences.
So maybe it only takes four or five genes to affect skin color. That's
certainly the sort of estimates people have made. And height. Oh, okay, maybe
there'll be some tweaking of 15 or 20 genes or something. But when you add it
all up, it's not that much.
Now, of course, the implications of this is small differences in the genetic
code can have big consequences for the appearance or the disease risk. I mean,
there's a single letter change, out of three billion letters, that can increase
your risk of Alzheimer's disease by 40-fold. So, little differences -- spelling
counts.
Krulwich: I don't quite understand. If you are looking at a black woman and
you're looking at a white woman, there's a drastic difference to your eye. Are
you saying that there is--
Lander: That's accounted for by a smallish number of genes, but that most of
the genetic variations that are going on within those populations are common to
both populations.
Krulwich: But from the gene's point of view, the two people who look so
different to your eye are remarkably similar, as far as the genes are
concerned.
Lander: Remarkably similar, and they both might be suffering from the same
predisposition to diabetes due to the same genetic variation, for example. They
might be suffering from other kinds of conditions that are exactly the same DNA
spelling difference. And it's only if you want to focus on those things that
are just skin deep that you can say, "Aha, the genes distribute differently in
the two populations." Otherwise, for most other things, the genes are
continuously present across all these populations.
So race is not a very helpful category to a geneticist, because it's focusing
on a fairly small number of genes that describe appearance. But if we're
talking about physiology, if we're talking about the 30,000 genes that run the
human symphony, that's a tapestry that weaves through every population. That's
why geneticists really don't think race is a terribly helpful concept.
Krulwich: And what about you and Arthur, your brother? What's the difference
between you and him?
Lander: He's a better cook, far better cook.
Krulwich: We're talking genetically.
Lander: Oh, genetics.
Krulwich: You come from the same parents, you're clearly not the same
people.
Lander: Well, 50 percent of our genes are identical, by descent. So the other
50 percent are as different as any two people on this planet, so I suppose
we're twice as close as any other two people on this planet, because we're
brothers.
Krulwich: So that's what we think of it -- that, as the closer you are in
relationship, the more you have in common, but there's this other whole
category, called everything else, which can vary.
Lander: Half of our chromosomes, we got exactly the same copy for that region.
The other half, it's as different as you and me.
Krulwich: And identical twins?
Lander: Identical twins, it's 100 percent of the chromosomes are the same. They
are genetically identical.
Krulwich: But they don't feel identical at all.
Lander: I didn't say they're identical. They're genetically identical. Whether
or not you're identical, well, that depends on your whole life experience, your
whole history. They're not identical people. But, at the genetic level, the
proteins are the same, the controls are the same. And it just reminds us the
outcomes can be quite different.
Krulwich: I want to do one thing before we go to the last category, about sex.
Men have something to celebrate, apparently. There is a headline that's good
for guys. Tell me the headline.
Lander: One of the cool things you can find by reading the genome sequence is
that the rate of mutation is different in sperm than in eggs. You can actually
see this by reading the genome story, by looking at how quickly certain things
are mutating if they happen to be on the Y chromosome versus on the X
chromosome. And by measuring that over 40 million years, we can find out that
about twice as much mutation is happening in sperm -- that is, in males passing
on their genes -- than in females. And that leads to a wonderful battle of the
sexes. The guys I know say, "Aha, men are responsible for two-thirds of all
evolutionary progress."
But, of course, women looking at that picture are equally well entitled to say
the guys are responsible for two-thirds of all the misery of genetic disease
that occurs through those mutations, too. Me, I'm going to be a conscientious
abstainer on this question.
Krulwich: Now when your group, the public group, decided to survey all the DNA
of a human being, which human beings did you survey?
Lander: So the genome that we sequenced, in fact, comes from about a dozen
different people.
Krulwich: A dozen? Were they fat ones, skinny ones, black ones, white
ones?
Lander: We don't know who they come from, because, in fact, what happened
was--
Krulwich: You don't know who they come from?
Lander: No. Part of the rules was that we don't know and they don't know. See,
ads were put in the papers, inviting people to sign up and donate DNA for the
Human Genome Project. But we got five or six times as many donors as we were
going to use.
Krulwich: What kind of ad? What sort of ad was it? "Please come downtown and
give us your blood," or "give us your cheek"--
Lander: Yes, yes. "There's an opportunity to donate DNA for a biomedical
research project." And then people had to receive a complete description of the
project and sign an informed consent document. The blood sample was taken. And
then--
Krulwich: What if a busload of very fat people came up and all nominated
themselves, and you had no skinnies? Then you would have the human genome of
the fat people.
Lander: Which differs by one tenth of a percent from anybody else.
Krulwich: Well, you don't know that going in.
Lander: Oh, we do. We have a really good sense of genetic variation. And it's
not a lot.
Krulwich: Now wait, I'm suspicious here. How do you-- You said you got a mix,
10 or 12 people.
Lander: Yeah.
Krulwich: Were they a mix of-- did they come from, some of them from Borneo and
some from America and some from--
Lander: So the genes from which most of the work was done come from Buffalo,
New York.
Krulwich: From Buffalo, New York?
Lander: Yes. It's mostly a guy from Buffalo and a woman from Buffalo. But
that's because the laboratory that was making--
Krulwich: An anonymous couple from Buffalo?
Lander: They're not a couple. They've never met. And we don't know who they
are. So we got a lot of the samples--
Krulwich: And they don't know who they are, except that
they--
Lander: The laboratory that prepared the large DNA libraries that were used was
a laboratory in Buffalo. And so they put an ad in the Buffalo newspapers, and
they got random volunteers from Buffalo, and they got about 20 of them. They
then erased all the labels and chose at random this sample and that sample and
that sample. So nobody knows who they are. We don't have any links back to who
they are, and that's deliberate.
But then to define all the human variation on top of it, we sequenced millions
and millions of DNA segments from a worldwide population of 24 people: Pacific
Islanders, Asians, Africans, Americans. And that defined more than
one-and-a-half million sites of genetic variation in the chromosomes.
Krulwich: So you feel you have a pretty good sample of humanity?
Lander: Oh, I think, about half of the common genetic variation that exists in
the human population is already represented in that data base of DNA
differences that we found amongst people. And what it does is, it reinforces
the message of how these differences are interwoven through the whole planet,
through the whole population.
Krulwich: And how many other creatures are we sequencing?
Lander: Oh, goodness, there's so much sequencing going on today. In terms of
large beasts, wow: vertebrates, mice are being sequenced right now, rats are
being sequenced. Two different kinds of puffer fish, one zebra fish, various
different types of worms, flies, a couple dozen fungi, bacteria, a couple more
plants going on. I mean, sequencing is such a great way to get a first look at
an organism. You'd be crazy not to do a little sequencing first.
Krulwich: One of the sort of happy futures that we're rolling towards is
customization. Tell me a little bit about what might be around the corner, in
that way. Three people have the same mistake, they go take the same medicine.
Two people get well, one doesn't. So what do you do for the third guy?
Lander: Well, what we're coming to understand is that three people who
supposedly have the same disease really may have it because of very different
reasons. You know, we call it all asthma, but it might be Asthma Type 1 and
Asthma Type 2 and Asthma Type 3. And the medicines might work very differently
in those people, because you're trying to fix something that's not broken in
the third person, and not fix the thing that is broken. Or you're giving
someone a medicine that's going to be broken down by their body in a different
way, maybe to something that's a toxic side product. Or maybe it'll just get
broken down four times as fast, and can't do its job.
We have different physiologies. And understanding those subtle differences in
physiology, whether it's the cause of the disease that's different or the
reaction to the medicine that's different, I think will be important in
matching the right drug to the right patient.
Krulwich: Well, in the same sense as there used to be Pan-American and TWA and
Eastern Airlines. They'd fly you everywhere. And there was Grand Union, and
there was the A&P, and they would sell you all the food. And there was NBC,
CBS, and ABC -- those were the broadcasters. Now medicine can narrow-cast.
Lander: Well, the hope is that medicine can narrow-cast. Of course, you can't
make it too narrow, because it's pretty expensive to develop drugs. And if we
make it so narrow that you can't actually afford to run the clinical trial,
we're not going to get medicines, either. I think the hope is that the broad
category of asthma will turn into four types of asthma, and that we'll be able
to develop drugs that have a much higher therapeutic index, much higher ability
to cure the disease in each of those four cases than before when we were just
trying to treat the general symptoms that were in common, but not the causes
that were different.
Krulwich: So Hayden [a boy profiled in the NOVA program "Cracking the Code of
Life" who has Tay Sachs, a fatal disease caused by a single-letter mutation],
like all human beings, has three point something billion chemicals inside the
DNA molecule, and one of the three billion--
Lander: One letter.
Krulwich: One letter.
Lander: One letter. It's I suppose understandable if you think about screws in
an airplane. There's an awful lot of screws in an airplane that don't matter if
you don't have them in the right place. Maybe your seat back will be a little
loose or something. But if it happens to be a screw on the propeller, it can
matter a lot. There are some positions that encode the information for unique
proteins, which you absolutely have to have. And if they're misspelled you
don't have this essential function, and you'll die.
Krulwich: Now that we have this sort of microscopic view, do you have the sense
that this is more unfair than we had previously realized? It seems amazing to
me that something so slight could result in such a huge difference.
Lander: Genetics is largely random. Every time your DNA is copied usually the
right letter is put in, but there's some very small probability the wrong
letter gets stuck in, and it's utterly random. Every time a baby is born, about
30 new mutations occur in the sperm and in the egg, giving rise to that baby.
It usually makes no difference because these 30 new mutations occur in places
that don't matter. But sometimes they occur in places that do matter, and
there's no rhyme or reason and no fairness in it. It's chance where they happen
to occur. And so, no, it's not fair.
Krulwich: The family says--and we asked them, you know, "What should have
happened in your case?" They say quite simply, "Well, we should have tested."
But they weren't Jewish, or they weren't obviously in the risk population. So
they say, "We really should have tested." In fact, everybody should test for
everything, because then we won't have situations like this. What are the
consequences of that logic?
Lander: Ideally we should understand everything that could go wrong and be able
to offer people the chance to test for whatever they want to. We're nowhere
near that today. We don't understand all the roles of all the genes. We don't
understand which misspellings really matter. And we also don't understand how
to explain to people enough about these choices for them to be able to make
intelligent decisions.
In some cases it's pretty clear. If you could know that you're at a very high
risk for having a baby with Tay Sachs, I think almost everybody would choose to
know. What if you're offered the chance to find out whether your baby might be
of high risk for Alzheimer's disease when he or she is 70? Would you want to
know? What if that risk is only 50 percent? What if you could be offered a
whole range of tests for whether or not you might have not the usual one
percent risk for some kind of a cancer but a four percent risk for that kind of
cancer?
I don't know quite what to do with it. It might be relevant information. It
might be the case that it's sensible to screen somebody twice as often if
they've got a four percent than a one percent risk. The challenge is going to
be two-fold. Understanding it as science and then understanding it as people.
How to deal with not certainties but probabilities, relative risks. We have got
a tremendous amount of work to do both in the laboratory and with the public to
get to the point where we know how to deal with this information and offer this
information in a way that's useful.
As a parent I just imagine what it would have been like if I could have been
offered a menu of 10,000 genetic tests to be done. See, there are changes in
many, many, many genes, and many of them don't mean anything; they're just
irrelevant changes. But you could get back a menu with all of them. Some of
them might matter and we can't tell. There will be tremendous anxiety in
knowing and there will be tremendous anxiety in not knowing. You might feel
irresponsible if you said, "I'm not going to find out." And you would feel
tremendous regret if something happened. But you might also feel just
tremendous pressure if you found out and doctors couldn't explain to you
whether it mattered or not.
We have opened a box here that has got a huge amount of valuable information.
It is the key for understanding disease and in the long run to curing disease.
But having opened it, we're also going to be very uncomfortable with that
information for some time to come. It would be immoral not to get this
information, because in it is the secret of cures. But it's going to be very
uncomfortable dealing with some of this information for the course of the next
century.
We obviously want to know about those things because by knowing the mechanism
for breast cancer or colon cancer or Alzheimer's, pharmaceutical companies can
try to work on cures. They can try to work on a drug that will slow down the
problem or prevent the problem. So we want that knowledge to fashion a cure,
but with it comes a certain cloud of uncertainty that can hang, and every
person has got to decide, "Do I want to find out about this uncertainty and
that uncertainty?"
Right now maybe we only have 10 or 12 uncertainties we can bestow upon people.
But fairly soon we may be able to offer hundreds of uncertainties, half which
you can do something about, have of which you can't.
The really great challenge for society is how we're going to stand by each
other, how we're going to say we all face exactly that same problem of having
potential knowledge about our genomes, but the potential knowledge is different
for each of us. Will we unite around the idea that we all are largely facing
the same set of issues? Or will we split apart into those people who have this
risk, that risk?
I worry a great deal about whether we're going to go down a path where we use
this genetic information to classify people, insure them differently, separate
people, or instead to unite people by saying, contrary to what they used to
say, "There's no perfect genome. None off us is particularly better or more
normal. We all have a wide range of variations. Those variations make us
spectacularly rich as a species, as in a population. But they also give us each
our own particular problems." I think the biggest choice ahead is how we're all
going to come to understand the ways in which we're genetically all the same
and genetically all different.
Krulwich: Last on this one, I guess I mentioned Justin, who is the son [who
also figures in the NOVA program, and whose genetic heritage predisposes him to
potentially developing a certain disease later in life)]. His way of handling
it is to say, "I just don't want to think about it right now." "I just don't
want to think about it right now" seems to me a perfectly acceptable position,
but it might be one of the hardest ones to have.
Lander: Oh, yeah. Saying that you don't want to think about a problem right now
is a perfectly valid response. If you really were mindful of all the potential
problems you could be completely paralyzed by worrying about everything.
Sometimes the happiest life might be lived by just saying, "Whatever happens
will happen. I'm going to get everything I can out of life and I'm not going to
worry about it." The hard part is where there are tests and diagnostics and
therapies that would let you change your risk. If we're talking about something
that you can't affect -- well, I don't go out and find out my risk for
Alzheimer's disease. I don't want to know because there's nothing I can do; it
will just make me worry or it will make me very depressed if I were to get bad
news.
But if I were to find out I was at special risk for colon cancer I would really
want to know because I really could do something about cutting that risk by
twofold or fourfold or tenfold. That's the hard part, is where sticking your
head in the sand really does come back to hurt you. At the moment there are a
limited number of cases where genetic knowledge can be translated into medical
action, but that will probably increase with time.
Krulwich: And my second last question for this whole shebang has to do with the
power of genes. I think a lot of people, particularly in a program that is
going one to two hours, get the impression that genes are our destiny to some
degree. You live with them and study them all the time. But how fragile are all
these predictions? Well, you have this gene, then consequently--
Lander: People often have this sense of genetic determinism -- that they're
nothing but their genes, that their genes contain their destiny written in the
DNA. This is nonsense. We know that there is a tremendous influence of
environment, of society, on outcome.
If you just think about the fact that the human gene pool hasn't significantly
changed in the last 5,000 years, and you notice how much society has changed
and opportunity has changed, you realize that our genes really can't determine
things. They can be influences, but for the longest time people said, "Well,
women are incapable of doing something biologically," or "Eastern Europeans
aren't smart enough for et cetera, et cetera." People looked to the genome to
justify their prejudices about what people could or couldn't do.
But when we look at it there is a huge range of what human societies have done,
how they have been organized, what opportunities people have had. That whole
range is encompassed within our genome. If that's the amount of limitation in
our genome that's okay; I'll live with that.
Krulwich: Do you have any sense, any spiritual sense, that what you've studied
reflects anything that isn't on the page?
Lander: Evolution is a pretty mysterious process. It works by randomness and
then selection. And I think we tremendously underestimate the power of random
experimentation. You think, oh, if I vary things, how could I ever get anything
sensible out of it? And when you look at the genome and you see the evidence of
churning and mixing and variation and duplication, you realize that this
completely undirected evolutionary force of variation generation is incredibly
powerful at invention, probably more powerful than design.
No committee of engineers getting together, no matter how smart, would have
come up with a human being. And yet evolution, by tinkering and getting it
wrong most of the time, but occasionally right, came up with a human being.
It's a pretty awesome process. We don't fully understand how it works. But it
is really humbling to look at a genome and see what that slow and steady
process of incremental improvement has wrought.
Krulwich: So if God is designing into this system, He may or She may or It may
have designed the evolutionary process, but there's nothing that you have seen
beyond the evolutionary process from your recent work?
Lander: The genome is awe-inspiring, but what the basis of that awe is, we
can't tell from that. We can see a remarkable history of experimentation, of
variation. But a lot of it looks like it's random churning and just saving a
couple of good things that worked. We can't see more than that in that. One of
the great things that--
Krulwich: You save a couple of good things and you save a couple of good
things, and it all adds up.
Lander: That's what they tell you, right? A little savings here, a little
savings there -- it sort of adds up. Well, there must be greater themes than
that, because evolution seems to have found ways to invent efficiently. See,
when it wants to make a new gene, it doesn't start from scratch. Maybe back in
the primordial ooze it would start from scratch, but then you had a lot of time
to work things out.
It seems, by looking at the human genome, that when evolution needs a new gene,
it waits for some existing gene to get randomly copied and then to slightly
work on it. You build out of existing parts. I think the secret of evolution is
that the genetic churning mechanism lets us make theme and variation and theme
and variation. And it turns out to be much more powerful than we ever imagined
to just make small variations on the theme. You get from a couple little
circuits to us.
Krulwich: So your recent emersion in this stuff just makes you think that
Darwin was smarter even than he even imagined?
Lander: This gives you a tremendous respect for life. It gives you respect for
the complexity of life, the innovation of life, and the tremendous connectivity
amongst all life on the planet. You come away from reading the genome
recognizing that we are so similar to everything else on the planet.
My take-home message from reading this genome is that we are such a piece of
every other living thing on this planet and every innovation in us, we didn't
really invent it. These were all things inherited from our ancestors, some of
which were yeasts, some of which were slugs. And we're walking around with all
these inventions that we got from our forebears, and making good use of it. But
we shouldn't be too proud about how wonderful the human is. Very little that's
human was invented in humans.
Krulwich: What is the intellectual excitement here [in studying the genome]? By
your description, once the DNA sequencing machines are invented, I just go
whoop, whishh, pishh! And then like I can take a nap, and then out comes the
answers.
Lander: You wish. It's just that, of course, since one is on the cutting edge
-- I guess in electronics they always call it "the bleeding edge" -- they know
what they're talking about, because nothing really is working as you expect.
All of the stuff we're doing will be working perfectly as soon as we're ready
to junk it. So we get the tremendous excitement about working with new ideas,
that are almost completely functional. And so you're constantly figuring out
why they're not completely functional. It's sort of like flying a very large
plane and repairing it while you're flying. It's a challenge, right?
The exhilarating thing about the genome project is we set ourselves the
challenge of trying to get this done really fast. If this wasn't important
stuff, you'd sit back and say, "Let's take a couple of years, and let's get it
all worked out just perfectly. We'll make sure it's humming. We'll work out
everything, and it will be flawless."
Of course, the information is too interesting. The insatiable hunger for this
information is pressing, and so you say, "Let's get the plane into the air, and
we'll work out the rest, 10 percent of the bugs, while we're on our ascent."
And it's an interesting challenge.
It attracts intellectual people, because it takes a lot of thinking to figure
out what's wrong. It attracts people who sort of love the exhilaration of
working together with other people. It attracts people who love the terror of
not knowing whether this is all going to fall together. And it also attracts
people who realize how much incredible information about medicine and evolution
is pouring out the back end, and that if we can only get it to flow perfectly,
we have a lifetime's worth of information to pour over, if we can just get it
to work.
Krulwich: And you didn't mention money.
Lander: Well, in fact, it flows from our computers straight onto the Internet
every 24 hours without patents, without any restrictions whatsoever. The one
commitment we made was that taking public money to do this, this was a kind of
basic knowledge that 10,000 medical scientists around the world could use every
day, 2,000 biotech companies, 30 pharmaceutical companies, and that there's no
way that we could do as much with this information all alone as the whole world
could do together.
And so the folks who did the genome project decided very early on that we would
just put our data on the Web, the information we got every single day, 24 hours
a day, and that way, anybody who is working on some brain degeneration or some
bowel disease could look at it, and see if they found something really useful.
So when we're writing up the paper about the human genome sequences, we're
doing right now, we have a tremendous pleasure. Most scientific papers you
speculate on how this stuff is going to be useful. We can write a whole section
about dozens and dozens and dozens of papers on different human diseases that
all have worked because of the sequences put out there.
I mean the folks at the genome centers around the world are the tremendous
heroes of this project, because everybody did this with no personal gain.
Everybody did this because they knew that they were doing the single most
important project they could be involved in in their life. And that they would
be proud to tell their grandchildren what they spent these years doing. And the
way we did it was incompatible with people seeking personal gain out of
particular genes. It wouldn't have worked unless everybody rode together.
Krulwich: This doesn't mean, yourself included, that people couldn't go across
the street and work for a private company?
Lander: You can go work for a private company. It means you can use this
information later, once it's been freely available to everybody to make
important discoveries. I have no objection to patents. I have no objection to
companies. Our feeling is that the basic information that the letters in the
cells were such fundamental infrastructure, such a foundation that that had to
be made available without any restrictions, and then companies can go off and
use our information, file patents, make millions of dollars. Scientists can do
that. Anybody at the genome center can go off and do research on it. But for
starters, the basic information has to be available to everybody. And then the
value can be added to it. I think that's the right solution for the
public.
Krulwich: Well, first of all, did it ever strike you as odd that there you
could patent something that we all have in almost all of our cells? Something
that's so natural as DNA, that it could become somebody's private property and
not mine?
Lander: Well, the patent office says, of course, that you can't patent the gene
in the human body. All you can do is get a patent on taking it out of the body
and putting it into a bacteria to produce a protein or using the DNA for a
diagnostic test. So they profess that you're not really patenting the human
gene.
Krulwich: You're taking the stuff out of us and putting it like a museum-like
pristine place, and then when it's isolated and pure --
Lander: You might use it to make a protein.
Krulwich: Yes. But it's still me.
Lander: Well, of course it's still you. But the patent office thinks once it's
outside of you, it's an invention; it's not you.
Krulwich: That's an odd idea.
Lander: Well, it's an odd idea, but when you get down to it, it's not
completely nutty to think about allowing some patents, because some of the
proteins in the body can be used as drugs as pharmaceuticals. If we didn't
allow some patenting, then a pharmaceutical company, thinking about spending
$100 million in order to develop this protein as a drug would say, "It's a
stupid idea. Somebody else could come along after we have a successful clinical
trial, not have invested the money, and just make the protein." So society
says, "All right. We'll grant the monopolies to these inventors."
Krulwich: Which lasts how long?
Lander: Twenty years. It used to be 17 years; it's now 20 years. "And because
of that, we're going to try to incent people to make inventions." It's not a
bad idea in principle. What bothers me about the patenting system is over the
last 10 years, we've been giving away patents for very trivial amounts of work.
I don't object to giving somebody that limited-time monopoly when they've
really invented a cure for a disease, some really important therapy. I do
object, because I think it's a crummy bargain, for society to giving a monopoly
when somebody has simply described a couple hundred letters of a gene, has no
idea what it does. Has no idea what use you could have in medicine. Because
what's going to happen is you've given away that precious monopoly to somebody
who's done a little bit of work, and then the people who want to come along,
and do a lot of work, to turn it into a therapy well they've got to go pay the
person who already owns it. I think it's a bad deal for society.
Krulwich: I want to make sure I understand this. So if this were back in the
1880's, and it was the mining [industry] -- I see a mountain. I think maybe
there's gold up there. I could go file a claim. I don't even have to go up the
hill. I don't have to dig a hole, just do it.
Lander: We went through this with the Homestead Act in the middle part of the
1800's. The government said, "We'll give you a big tract of land, if you will
work the land for three years." That was a great bargain, because we gave land
and people developed its economic uses. If the Homestead Act instead said,
"We'll give you land that all you have to do is walk the boundary," we would
have accomplished nothing. That's the difference.
If you've got to work your claim, if you've really got to by the sweat of your
brow add value for society, I don't object to these limited-time monopolies.
But if we're giving away the store for effectively no work, I think society is
getting the short end of the bargain.
Krulwich: Let me see what you get. If you haven't done a whole lot, I guess you
want the people to do some serious science, some research, some benchmark,
actually manipulate these things?
Lander: I want, in order to get a patent, that someone do something that we
would all really call useful. Not just that a patent examiner might say is
technically satisfying the condition of utility, but that a person on the
street would acknowledge, "That is useful."
Krulwich: Were companies patenting segment after segment after segment, doing
almost nothing at all to get the patent?
Lander: Oh, yeah. One could write automated programs to file patents on the
automated sequences you generated.
Krulwich: No!
Lander: Oh, yes. Sure. Why not?
Krulwich: You'd have a robot do the science--
Lander: You'd have a robot write the patent.
Krulwich: A robot do the research?
Lander: Yeah.
Krulwich: And what would you do; you'd just become a landlord?
Lander: You got to put the stamp on it.
Krulwich: So that's stopped, right? I mean the patent office changed its
mind.
Lander: Well, the patent office has been slowly ratcheting up the standards. I
honestly don't know if there really is an appropriate level right now. They're
certainly imposing more and more of an obligation on a would-be inventor to say
that they've done something really meaningful....
Krulwich: Let's say now I have to worry, though, that if I work this particular
set that some landlord is going to call me up and say, "I own this. You have to
pay me to work here." Isn't that what the landlord does?
Lander: Well, you mostly have to worry if you're a scientist in a company, who
is trying to make some important therapeutic, some cure, based on that segment.
If you're a research scientist, just doing research, in principle the other
company could call you up and tell you to cease and desist. In practice, of
course, they're not going to bother. In fact, they're happy you're doing it,
because you're adding value to their property.
Krulwich: Oh, I got it. Okay.
Lander: You know, you're putting a hotel on their square.
Krulwich: So let's change the argument. I'm a company trying to do work on
this, this, and this rung of the ladder, because I think that I can maybe
develop a cure for cancer right here, just for the sake of argument. But, of
course, I have to worry that somebody owns this thing.
Lander: Oh, you have to worry a lot that this region here that you're working
on that might cure cancer has already been patented by somebody else and that
patent filing is not public. And so you're living with the shadow that all of
your work may go for naught.
Krulwich: Because one day the phone rings, and says, "Sorry, you can't work
here. Get off my territory."
Lander: That's right.
Krulwich: Or "You can work here, but I'm going to charge $100,000 a week," or
"You can work here, and I'll charge you a nickel, but I want 50 percent of
whatever you discover."
Lander: And the problem here is even worse, because many companies don't start
the work whenever there's a cloud over who owns that. If there's uncertainty,
companies would rather be working some place where they don't have uncertainty,
and, therefore, I think work doesn't get done, because of the confusion over
who owns stuff.
Krulwich: Is there any place on the genome that is safe to work on, that's
unclouded by legal uncertainty?
Lander: No. We're just not aware necessarily of what anybody has done, and so
there's no place concrete that's unclouded.
Krulwich: So one argument could be that this patent system that we have
frustrates our very basic desire which is to get medicines to people
quickly.
Lander: I agree.
Krulwich: Very briefly, I need you to help me describe what the business of
these businesses is? In the case of Celera and some of these other companies,
they don't actually seem to be in the landlord business. At least to hear them
describe it, they're in some other kind of business. What is that
business?
Lander: It's interesting. The companies that are involved in sequencing the
genome, and there are really three that have popped up all through the course
of the 1990's, could either be seen to be in the landlord business, holding
patents on genes, and waving them around when people want to come settle on
that gene, or they could be seen as being in the database business. That is
having some computer database, where any researcher who wants to gain access to
information about the genome could pay a subscription fee and get the data,
plus some tools to study it.
Krulwich: Would it tell you the names of the universities that are working that
part of the territory, and the names of the professors and their telephone
numbers and the articles they have published? And what--
Lander: It depends whether they put that into the database. In the first
instance, they could just tell you the letters. Maybe they might also tell you,
"We think there are genes here." Maybe they might also tell you, "We think this
gene is expressed in your intestines." And maybe they would tell you, "Here're
all the papers that have ever been written about this gene." That's valuable
information. There are databases of geography of the United States that will
tell you, you know, where the cities are, where the buildings are, who lives in
what buildings. These are useful databases, and I have no objection to having
databases available.
But the raw data, for example, of who is in the phone book, that should be
available to everybody. But if you want to build a commercial database, that
adds more value and aggregates together those people who live in the same
neighborhood and also, you know, buy books from Amazon.com and all these other
-- more power to you, that's great.
Krulwich: Finally, what was the most fun that you have had since this whole
thing began? Was it standing there at the White House with all these prime
ministers?
Lander: No.
Krulwich: Was it the toast you gave, which we have, which is beautiful, where
you're looking at the people who helped you get to the billionth base pair,
when you had the sort of daddy look on? Or what was it? Was there some
intellectual thing?
Lander: I went into biology because I have the sense that it was one place
where you could really share your pleasures, where you could really share what
you were doing as part of a whole community. That's what I got out of the
genome project.
We pulled together through some really challenging, really difficult times, and
we did it. We did what we set out to do, and it's something we're all going to
tell our grandchildren about. And it's pretty wonderful. That feeling of having
pulled together with other people and accomplished something that matters, let
alone something that I think will matter for hundreds of years, that's a pretty
wonderful feeling. I couldn't have asked for anything better in all the time I
was wandering around figuring out what to do with my career. It's a tremendous
gift.
Krulwich: Was this your Mark Spitz U.S. Hockey Team moment, after which there
will never be as high a high?
Lander: Oh, golly, no. I think this was a very special time in its own way. It
was also a very stressful time, it was a challenging time. But I think it was
special. But, you know, the fun is just going to start now. I think it's a
different kind of fun. I think we have finally gotten to the other side of this
massive genome. We saw this vast hill, this vast mountain standing in front of
us 15 years ago. Now here we are. We've gotten over to the other side. And
there's this huge new land to explore.
So it was a tremendous feeling to have been part of an expedition going up this
mountain and coming down the other side. And that probably won't be replaced by
any other experience. But, boy, the experience of seeing this huge valley now
just spread out in front of us! That's going to have years and years of fun
ahead. I'm really looking forward to that part, too.
Interviews:
Collins |
Lander |
Venter
Photo: WGBH/NOVA.
Watch the Program Here |
Our Genetic Future (A Survey)
Manipulating Genes: How Much is Too Much? |
Understanding Heredity
Explore a Stretch of Code |
Nature vs Nurture Revisited
Sequence for Yourself |
Journey into DNA |
Meet the Decoders
Resources |
Update to Program |
Teacher's Guide |
Transcript
Site Map |
Cracking the Code of Life Home
Editor's Picks |
Previous Sites |
Join Us/E-mail |
TV/Web Schedule |
About NOVA
Watch NOVAs online |
Teachers |
Site Map |
Shop |
Search |
To Print
PBS Online |
NOVA Online |
WGBH
© | Updated April 2001
|
|
|