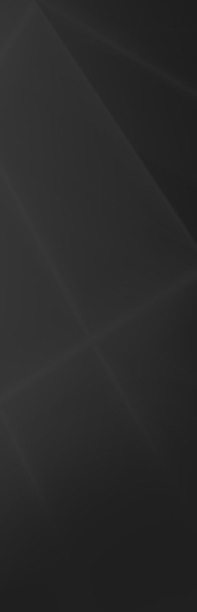
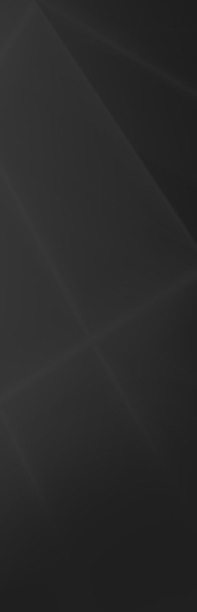
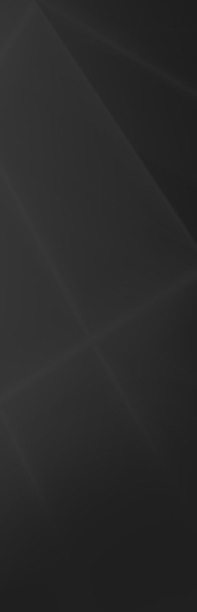
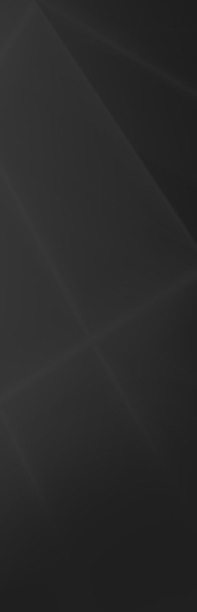
The Elegant Universe
Eleven dimensions, parallel universes, and a world made out of strings. It's not science fiction, it's string theory. Aired 7/11, 7/18, and 7/25, 2012 on PBS Aired 7/11, 7/18, and 7/25, 2012 on PBS
- Originally aired 10.28.03
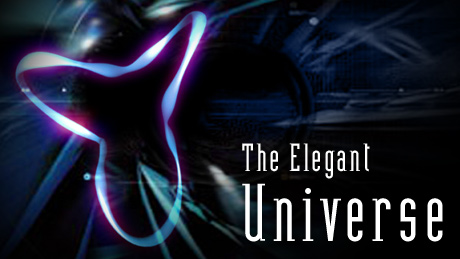
Program Description
Preview
Full Program
Full program available for streaming through
Watch Online
Full program available
Soon
The Elegant Universe: Pt 1
Einstein's Dream: Combining the laws of the universe in one theory that explains it all is the Holy Grail of physics. Airing July 11, 2012 at 9 pm on PBS Aired July 11, 2012 on PBS
- Originally aired 10.28.03
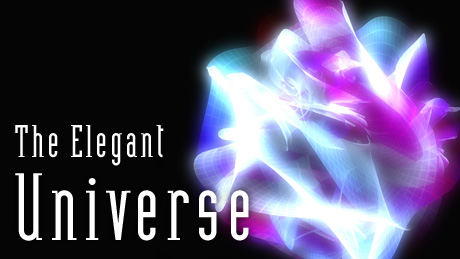
Program Description
Transcript
The Elegant Universe: Part 1
PBS Airdate: October 28, 2003
NARRATOR: Now, on NOVA, take a thrill ride into a world stranger than science fiction, where you play the game, by breaking some rules, where a new view of the universe, pushes you beyond the limits of your wildest imagination. This is the world of string theory, a way of describing every force and all matter from an atom to earth, to the end of the galaxies—from the birth of time to its final tick—in a single theory, a theory of everything. Our guide to this brave new world is Brian Greene, the bestselling author and physicist.
BRIAN GREENE (Columbia University): And no matter how many times I come here, I never seem to get used to it.
NARRATOR: Can he help us solve the greatest puzzle of modern physics—that our understanding of the universe is based on two sets of laws, that don't agree?
NARRATOR: Resolving that contradiction eluded even Einstein, who made it his final quest. After decades, we may finally be on the verge of a breakthrough. The solution is strings, tiny bits of energy vibrating like the strings on a cello, a cosmic symphony at the heart of all reality. But it comes at a price: parallel universes and 11 dimensions, most of which you've never seen.
BRIAN GREENE: We really may live in a universe with more dimensions than meet the eye.
AMANDA PEET (University of Toronto): People who have said that there were extra dimensions of space have been labeled crackpots, or people who are bananas.
NARRATOR: A mirage of science and mathematics or the ultimate theory of everything?
S. JAMES GATES, JR. (University of Maryland): If string theory fails to provide a testable prediction, then nobody should believe it.
SHELDON LEE GLASHOW (Boston University): Is that a theory of physics, or a philosophy?
BRIAN GREENE: One thing that is certain is that string theory is already showing us that the universe may be a lot stranger than any of us ever imagined.
NARRATOR: Coming up tonight...it all started with an apple.
BRIAN GREENE: The triumph of Newton's equations come from the quest to understand the planets and the stars.
NARRATOR: And we've come a long way since.
BRIAN GREENE: Einstein gave the world a new picture for what the force of gravity actually is.
NARRATOR: Where he left off, string theorists now dare to go. But how close are they to fulfilling Einstein's dream? Watch The Elegant Universe right now.
BRIAN GREENE: Fifty years ago, this house was the scene of one of the greatest mysteries of modern science, a mystery so profound that today thousands of scientists on the cutting edge of physics are still trying to solve it.
Albert Einstein spent his last two decades in this modest home in Princeton, New Jersey. And in his second floor study Einstein relentlessly sought a single theory so powerful it would describe all the workings of the universe. Even as he neared the end of his life Einstein kept a notepad close at hand, furiously trying to come up with the equations for what would come to be known as the "Theory of Everything."
Convinced he was on the verge of the most important discovery in the history of science, Einstein ran out of time, his dream unfulfilled.
Now, almost a half century later, Einstein's goal of unification—combining all the laws of the universe in one, all-encompassing theory—has become the Holy Grail of modern physics. And we think we may at last achieve Einstein's dream with a new and radical set of ideas called "string theory."
But if this revolutionary theory is right, we're in for quite a shock. String theory says we may be living in a universe where reality meets science fiction—a universe of eleven dimensions with parallel universes right next door—an elegant universe composed entirely of the music of strings.
But for all its ambition, the basic idea of string theory is surprisingly simple. It says that everything in the universe, from the tiniest particle to the most distant star is made from one kind of ingredient—unimaginably small vibrating strands of energy called strings.
Just as the strings of a cello can give rise to a rich variety of musical notes, the tiny strings in string theory vibrate in a multitude of different ways making up all the constituents of nature. In other words, the universe is like a grand cosmic symphony resonating with all the various notes these tiny vibrating strands of energy can play.
String theory is still in its infancy, but it's already revealing a radically new picture of the universe, one that is both strange and beautiful. But what makes us think we can understand all the complexity of the universe, let alone reduce it to a single "Theory of Everything?"
We have R mu nu, minus a half g mu nu R—you remember how this goes—equals eight Pi G T mu nu...comes from varying the Einstein-Hilbert action, and we get the field equations and this term. You remember what this is called?
DOG BARKS
No that's the scalar curvature. This is the ricci tensor. Have you been studying this at all?
No matter how hard you try, you can't teach physics to a dog. Their brains just aren't wired to grasp it. But what about us? How do we know that we're wired to comprehend the deepest laws of the universe? Well, physicists today are confident that we are, and we're picking up where Einstein left off in his quest for unification.
Unification would be the formulation of a law that describes, perhaps, everything in the known universe from one single idea, one master equation. And we think that there might be this master equation, because throughout the course of the last 200 years or so, our understanding of the universe has given us a variety of explanations that are all pointing towards one spot. They seem to all be converging on one nugget of an idea that we're still trying to find.
STEVEN WEINBERG (University of Texas at Austin): Unification is where it's at. Unification is what we're trying to accomplish. The whole aim of fundamental physics is to see more and more of the world's phenomena in terms of fewer and fewer and simpler and simpler principles.
MICHAEL B. GREEN (University of Cambridge): We feel, as physicists, that if we can explain a wide number of phenomena in a very simple manner, that that's somehow progress. There is almost an emotional aspect to the way in which the great theories in physics sort of encompass a wide variety of apparently different physical phenomena. So this idea that we should be aiming to unify our understanding is inherent, essentially, to the whole way in which this kind of science progresses.
BRIAN GREENE: And long before Einstein, the quest for unification began with the most famous accident in the history of science. As the story goes, one day in 1665, a young man was sitting under a tree when, all of a sudden, he saw an apple fall from above. And with the fall of that apple, Isaac Newton revolutionized our picture of the universe.
In an audacious proposal for his time, Newton proclaimed that the force pulling apples to the ground and the force keeping the moon in orbit around the earth were actually one and the same. In one fell swoop, Newton unified the heavens and the earth in a single theory he called gravity.
STEVEN WEINBERG: The unification of the celestial with the terrestrial—that the same laws that govern the planets in their motions govern the tides and the falling of fruit here on earth—it was a fantastic unification of our picture of nature.
BRIAN GREENE: Gravity was the first force to be understood scientifically, though three more would eventually follow. And, although Newton discovered his law of gravity more than 300 years ago, his equations describing this force make such accurate predictions that we still make use of them today. In fact scientists needed nothing more than Newton's equations to plot the course of a rocket that landed men on the moon.
Yet there was a problem. While his laws described the strength of gravity with great accuracy, Newton was harboring an embarrassing secret: he had no idea how gravity actually works.
For nearly 250 years, scientists were content to look the other way when confronted with this mystery. But in the early 1900s, an unknown clerk working in the Swiss patent office would change all that. While reviewing patent applications, Albert Einstein was also pondering the behavior of light. And little did Einstein know that his musings on light would lead him to solve Newton's mystery of what gravity is.
At the age of 26, Einstein made a startling discovery: that the velocity of light is a kind of cosmic speed limit, a speed that nothing in the universe can exceed. But no sooner had the young Einstein published this idea than he found himself squaring off with the father of gravity.
The trouble was, the idea that nothing can go faster than the speed of light flew in the face of Newton's picture of gravity. To understand this conflict, we have to run a few experiments. And to begin with, let's create a cosmic catastrophe.
Imagine that all of a sudden, and without any warning, the sun vaporizes and completely disappears. Now, let's replay that catastrophe and see what effect it would have on the planets according to Newton.
Newton's theory predicts that with the destruction of the sun, the planets would immediately fly out of their orbits careening off into space. In other words, Newton thought that gravity was a force that acts instantaneously across any distance. And so we would immediately feel the effect of the sun's destruction.
But Einstein saw a big problem with Newton's theory, a problem that arose from his work with light. Einstein knew light doesn't travel instantaneously. In fact, it takes eight minutes for the sun's rays to travel the 93 million miles to the earth. And since he had shown that nothing, not even gravity, can travel faster than light, how could the earth be released from orbit before the darkness resulting from the sun's disappearance reached our eyes?
To the young upstart from the Swiss patent office anything outrunning light was impossible, and that meant the 250-year old Newtonian picture of gravity was wrong.
S. JAMES GATES, JR.: If Newton is wrong, then why do the planets stay up? Because remember, the triumph of Newton's equations come from the quest to understand the planets and the stars, and particularly the problem of why the planets have the orbits that they do. And with Newton's equations you could calculate the way that the planets would move. Einstein's got to resolve this dilemma.
BRIAN GREENE: In his late twenties, Einstein had to come up with a new picture of the universe in which gravity does not exceed the cosmic speed limit. Still working his day job in the patent office, Einstein embarked on a solitary quest to solve this mystery. After nearly ten years of wracking his brain he found the answer in a new kind of unification.
PETER GALISON (Harvard University): Einstein came to think of the three dimensions of space and the single dimension of time as bound together in a single fabric of "space-time." It was his hope that by understanding the geometry of this four-dimensional fabric of space-time, that he could simply talk about things moving along surfaces in this space-time fabric.
BRIAN GREENE: Like the surface of a trampoline, this unified fabric is warped and stretched by heavy objects like planets and stars. And it's this warping or curving of space-time that creates what we feel as gravity.
A planet like the earth is kept in orbit, not because the sun reaches out and instantaneously grabs hold of it, as in Newton's theory, but simply because it follows curves in the spatial fabric caused by the sun's presence. So, with this new understanding of gravity, let's rerun the cosmic catastrophe. Let's see what happens now if the sun disappears.
The gravitational disturbance that results will form a wave that travels across the spatial fabric in much the same way that a pebble dropped into a pond makes ripples that travel across the surface of the water. So we wouldn't feel a change in our orbit around the sun until this wave reached the earth.
What's more, Einstein calculated that these ripples of gravity travel at exactly the speed of light. And so, with this new approach, Einstein resolved the conflict with Newton over how fast gravity travels. And more than that, Einstein gave the world a new picture for what the force of gravity actually is: it's warps and curves in the fabric of space and time.
Einstein called this new picture of gravity "General Relativity," and within a few short years Albert Einstein became a household name.
S. JAMES GATES, JR.: Einstein was like a rock star in his day. He was one of the most widely known and recognizable figures alive. He and perhaps Charlie Chaplin were the reigning kings of the popular media.
MARCIA BARTUSIAK (Author): People followed his work. And they were anticipating...because of this wonderful thing he had done with general relativity, this recasting the laws of gravity out of his head...there was a thought he could do it again, and they, you know, people want to be in on that.
BRIAN GREENE: Despite all that he had achieved Einstein wasn't satisfied. He immediately set his sights on an even grander goal, the unification of his new picture of gravity with the only other force known at the time, electromagnetism.
Now electromagnetism is a force that had itself been unified only a few decades earlier. In the mid-1800s, electricity and magnetism were sparking scientists' interest. These two forces seemed to share a curious relationship that inventors like Samuel Morse were taking advantage of in newfangled devices, such as the telegraph.
An electrical pulse sent through a telegraph wire to a magnet thousands of miles away produced the familiar dots and dashes of Morse code that allowed messages to be transmitted across the continent in a fraction of a second. Although the telegraph was a sensation, the fundamental science driving it remained something of a mystery.
But to a Scottish scientist named James Clark Maxwell, the relationship between electricity and magnetism was so obvious in nature that it demanded unification.
If you've ever been on top of a mountain during a thunderstorm you'll get the idea of how electricity and magnetism are closely related. When a stream of electrically charged particles flows, like in a bolt of lightning, it creates a magnetic field. And you can see evidence of this on a compass.
Obsessed with this relationship, the Scot was determined to explain the connection between electricity and magnetism in the language of mathematics. Casting new light on the subject, Maxwell devised a set of four elegant mathematical equations that unified electricity and magnetism in a single force called "electromagnetism." And like Isaac Newton's before him, Maxwell's unification took science a step closer to cracking the code of the universe.
JOSEPH POLCHINSKI (University of California, Santa Barbara): That was really the remarkable thing, that these different phenomena were really connected in this way. And it's another example of diverse phenomena coming from a single underlying building block or a single underlying principle.
WALTER H.G. LEWIN (Massachusetts Institute of Technology): Imagine that everything that you can think of which has to do with electricity and magnetism can all be written in four very simple equations. Isn't that incredible? Isn't that amazing? I call that elegant.
PETER GALISON: Einstein thought that this was one of the triumphant moments of all of physics and admired Maxwell hugely for what he had done.
BRIAN GREENE: About 50 years after Maxwell unified electricity and magnetism, Einstein was confident that if he could unify his new theory of gravity with Maxwell's electromagnetism, he'd be able to formulate a master equation that could describe everything, the entire universe.
S. JAMES GATES, JR.: Einstein clearly believes that the universe has an overall grand and beautiful pattern to the way that it works. So to answer your question, why was he looking for the unification? I think the answer is simply that Einstein is one of those physicists who really wants to know the mind of God, which means the entire picture.
BRIAN GREENE: Today, this is the goal of string theory: to unify our understanding of everything from the birth of the universe to the majestic swirl of galaxies in just one set of principles, one master equation. Newton had unified the heavens and the earth in a theory of gravity. Maxwell had unified electricity and magnetism. Einstein reasoned all that remained to build a "Theory of Everything"—a single theory that could encompass all the laws of the universe—was to merge his new picture of gravity with electromagnetism.
AMANDA PEET: He certainly had motivation. Probably one of them might have been aesthetics, or this quest to simplify. Another one might have been just the physical fact that it seems like the speed of gravity is equal to the speed of light. So if they both go at the same speed, then maybe that's an indication of some underlying symmetry.
BRIAN GREENE: But as Einstein began trying to unite gravity and electromagnetism he would find that the difference in strength between these two forces would outweigh their similarities.
Let me show you what I mean. We tend to think that gravity is a powerful force. After all, it's the force that, right now, is anchoring me to this ledge. But compared to electromagnetism, it's actually terribly feeble. In fact, there's a simple little test to show this. Imagine that I was to leap from this rather tall building. Actually, let's not just imagine it. Let's do it. You'll see what I mean.
Now, of course, I really should have been flattened. But the important question is: what kept me from crashing through the sidewalk and hurtling right down to the center of the earth? Well, strange as it sounds, the answer is electromagnetism.
Everything we can see, from you and me to the sidewalk, is made of tiny bits of matter called atoms. And the outer shell of every atom contains a negative electrical charge. So when my atoms collide with the atoms in the cement these electrical charges repel each other with such strength that just a little piece of sidewalk can resist the entire Earth's gravity and stop me from falling. In fact the electromagnetic force is billions and billions of times stronger than gravity.
NIMA ARKANI-HAMED (Harvard University): That seems a little strange, because gravity keeps our feet to the ground, it keeps the earth going around the sun. But, in actual fact, it manages to do that only because it acts on huge enormous conglomerates of matter, you know—you, me, the earth, the sun—but really at the level of individual atoms, gravity is a really incredibly feeble tiny force.
BRIAN GREENE: It would be an uphill battle for Einstein to unify these two forces of wildly different strengths. And to make matters worse, barely had he begun before sweeping changes in the world of physics would leave him behind.
STEVEN WEINBERG: Einstein had achieved so much in the years up to about 1920, that he naturally expected that he could go on by playing the same theoretical games and go on achieving great things. And he couldn't. Nature revealed itself in other ways in the 1920s and 1930s, and the particular tricks and tools that Einstein had at his disposal had been so fabulously successful, just weren't applicable anymore.
BRIAN GREENE: You see, in the 1920s a group of young scientists stole the spotlight from Einstein when they came up with an outlandish new way of thinking about physics.
Their vision of the universe was so strange, it makes science fiction look tame, and it turned Einstein's quest for unification on its head. Led by Danish physicist Niels Bohr, these scientists were uncovering an entirely new realm of the universe.
Atoms, long thought to be the smallest constituents of nature, were found to consist of even smaller particles: the now-familiar nucleus of protons and neutrons orbited by electrons. And the theories of Einstein and Maxwell were useless at explaining the bizarre way these tiny bits of matter interact with each other inside the atom.
PETER GALISON: There was a tremendous mystery about how to account for all this, how to account for what was happening to the nucleus as the atom began to be pried apart in different ways. And the old theories were totally inadequate to the task of explaining them. Gravity was irrelevant. It was far too weak. And electricity and magnetism was not sufficient.
BRIAN GREENE: Without a theory to explain this strange new world, these scientists were lost in an unfamiliar atomic territory looking for any recognizable landmarks.
Then, in the late 1920s, all that changed. During those years, physicists developed a new theory called "quantum mechanics," and it was able to describe the microscopic realm with great success. But here's the thing: quantum mechanics was so radical a theory that it completely shattered all previous ways of looking at the universe.
Einstein's theories demand that the universe is orderly and predictable, but Niels Bohr disagreed. He and his colleagues proclaimed that at the scale of atoms and particles, the world is a game of chance. At the atomic or quantum level, uncertainty rules. The best you can do, according to quantum mechanics, is predict the chance or probability of one outcome or another. And this strange idea opened the door to an unsettling new picture of reality.
It was so unsettling that if the bizarre features of quantum mechanics were noticeable in our everyday world, like they are here in the Quantum Café, you might think you'd lost your mind.
WALTER H.G. LEWIN: The laws in the quantum world are very different from the laws that we are used to. Our daily experiences are totally different from anything that you would see in the quantum world. The quantum world is crazy. It's probably the best way to put it: it's a crazy world.
BRIAN GREENE: For nearly 80 years, quantum mechanics has successfully claimed that the strange and bizarre are typical of how our universe actually behaves on extremely small scales. At the scale of everyday life, we don't directly experience the weirdness of quantum mechanics. But here in the Quantum Café, big, everyday things sometimes behave as if they were microscopically tiny. And no matter how many times I come here, I never seem to get used to it.
I'll have an orange juice, please.
BARTENDER: I'll try.
BRIAN GREENE: "I'll try," she says. You see, they're not used to people placing definite orders here in the Quantum Café, because here everything is ruled by chance. While I'd like an orange juice, there is only a particular probability that I'll actually get one.
And there's no reason to be disappointed with one particular outcome or another, because quantum mechanics suggests that each of the possibilities like getting a yellow juice or a red juice may actually happen. They just happen to happen in universes that are parallel to ours, universes that seem as real to their inhabitants as our universe seems to us.
WALTER H.G. LEWIN: If there are a thousand possibilities, and quantum mechanics cannot, with certainty, say which of the thousand it will be, then all thousand will happen. Yeah, you can laugh at it and say, "Well, that has to be wrong." But there are so many other things in physics which—at the time that people came up with—had to be wrong, but it wasn't. Have to be a little careful, I think, before you say this is clearly wrong.
BRIAN GREENE: And even in our own universe, quantum mechanics says there's a chance that things we'd ordinarily think of as impossible can actually happen. For example there's a chance that particles can pass right through walls or barriers that seem impenetrable to you or me. There's even a chance that I could pass through something solid, like a wall. Now, quantum calculations do show that the probability for this to happen in the everyday world is so small that I'd need to continue walking into the wall for nearly an eternity before having a reasonable chance of succeeding. But here, these kinds of things happen all the time.
EDWARD FARHI (Massachusetts Institute of Technology): You have to learn to abandon those assumptions that you have about the world in order to understand quantum mechanics. In my gut, in my belly, do I feel like I have a deep intuitive understanding of quantum mechanics? No.
BRIAN GREENE: And neither did Einstein. He never lost faith that the universe behaves in a certain and predictable way. The idea that all we can do is calculate the odds that things will turn out one way or another was something Einstein deeply resisted.
MICHAEL DUFF (University of Michigan): Quantum mechanics says that you can't know for certain the outcome of any experiment; you can only assign a certain probability to the outcome of any experiment. And this, Einstein disliked intensely. He used to say "God does not throw dice."
BRIAN GREENE: Yet, experiment after experiment showed Einstein was wrong and that quantum mechanics really does describe how the world works at the subatomic level.
WALTER H.G. LEWIN: So quantum mechanics is not a luxury, something that you can do without. I mean why is water the way it is? Why does light go straight through water? Why is it transparent? Why are other things not transparent? How do molecules form? Why are they reacting the way they react? The moment that you want to understand anything at an atomic level, as non-intuitive as it is, at that moment, you can only make progress with quantum mechanics.
EDWARD FARHI: Quantum mechanics is fantastically accurate. There has never been a prediction of quantum mechanics that has contradicted an observation, never.
BRIAN GREENE: By the 1930s, Einstein's quest for unification was floundering, while quantum mechanics was unlocking the secrets of the atom. Scientists found that gravity and electromagnetism are not the only forces ruling the universe. Probing the structure of the atom, they discovered two more forces.
One, dubbed the "strong nuclear force," acts like a super-glue, holding the nucleus of every atom together, binding protons to neutrons. And the other, called the "weak nuclear force," allows neutrons to turn into protons, giving off radiation in the process.
At the quantum level, the force we're most familiar with, gravity, was completely overshadowed by electromagnetism and these two new forces.
Now, the strong and weak forces may seem obscure, but in one sense at least, we're all very much aware of their power. At 5:29 on the morning of July 16th, 1945, that power was revealed by an act that would change the course of history. In the middle of the desert, in New Mexico, at the top of a steel tower about a hundred feet above the top of this monument, the first atomic bomb was detonated.
It was only about five feet across, but that bomb packed a punch equivalent to about twenty thousand tons of TNT. With that powerful explosion, scientists unleashed the strong nuclear force, the force that keeps neutrons and protons tightly glued together inside the nucleus of an atom. By breaking the bonds of that glue and splitting the atom apart, vast, truly unbelievable amounts of destructive energy were released.
We can still detect remnants of that explosion through the other nuclear force, the weak nuclear force, because it's responsible for radioactivity. And today, more than 50 years later, the radiation levels around here are still about 10 times higher than normal.
So, although in comparison to electromagnetism and gravity the nuclear forces act over very small scales, their impact on everyday life is every bit as profound.
But what about gravity? Einstein's general relativity? Where does that fit in at the quantum level? Quantum mechanics tells us how all of nature's forces work in the microscopic realm except for the force of gravity. Absolutely no one could figure out how gravity operates when you get down to the size of atoms and subatomic particles. That is, no one could figure out how to put general relativity and quantum mechanics together into one package.
For decades, every attempt to describe the force of gravity in the same language as the other forces—the language of quantum mechanics—has met with disaster.
S. JAMES GATES, JR.: You try to put those two pieces of mathematics together, they do not coexist peacefully.
STEVEN WEINBERG: You get answers that the probabilities of the event you're looking at are infinite. Nonsense, it's not profound, it's just nonsense.
NIMA ARKANI-HAMED: It's very ironic because it was the first force to actually be understood in some decent quantitative way, but, but, but it still remains split off and very different from, from the other ones.
S. JAMES GATES, JR.: The laws of nature are supposed to apply everywhere. So if Einstein's laws are supposed to apply everywhere, and the laws of quantum mechanics are supposed to apply everywhere, well you can't have two separate everywheres.
BRIAN GREENE: In 1933, after fleeing Nazi Germany, Einstein settled in Princeton, New Jersey. Working in solitude, he stubbornly continued the quest he had begun more than a decade earlier, to unite gravity and electromagnetism. Every few years, headlines appeared, proclaiming Einstein was on the verge of success. But most of his colleagues believed his quest was misguided and that his best days were already behind him.
STEVEN WEINBERG: Einstein, in his later years, got rather detached from
the work of physics in general and, and stopped reading people's papers. I
didn't even think he knew there was such a thing as the weak nuclear force. He
didn't pay attention to those things. He kept working on the same problem that
he had started working on as a younger man.
S JAMES GATES, JR.: When the community of theoretical physicists begins to probe the atom, Einstein very definitely gets left out of the picture. He, in some sense, chooses not to look at the physics coming from these experiments. That means that the laws of quantum mechanics play no role in his sort of further investigations. He's thought to be this doddering, sympathetic old figure who led an earlier revolution but somehow fell out of it.
STEVEN WEINBERG: It is as if a general who was a master of horse cavalry, who has achieved great things as a commander at the beginning of the First World War, would try to bring mounted cavalry into play against the barbwire trenches and machines guns of the other side.
BRIAN GREENE: Albert Einstein died on April 18, 1955. And for many years it seemed that Einstein's dream of unifying the forces in a single theory died with him.
S. JAMES GATES, JR.: So the quest for unification becomes a backwater of physics. By the time of Einstein's death in the '50s, almost no serious physicists are engaged in this quest for unification.
RIGHT SIDE BRIAN GREENE: In the years since, physics split into two separate camps: one that uses general relativity to study big and heavy objects, things like stars, galaxies and the universe as a whole...
LEFT SIDE BRIAN GREENE: ...and another that uses quantum mechanics to study the tiniest of objects, like atoms and particles. This has been kind of like having two families that just cannot get along and never talk to each other...
RIGHT SIDE BRIAN GREENE: ...living under the same roof.
LEFT SIDE BRIAN GREENE: There just seemed to be no way to combine quantum mechanics...
RIGHT SIDE BRIAN GREENE: ...and general relativity in a single theory that could describe the universe on all scales.
BRIAN GREENE: Now, in spite of this, we've made tremendous progress in understanding the universe. But there's a catch: there are strange realms of the cosmos that will never be fully understood until we find a unified theory.
And nowhere is this more evident than in the depths of a black hole. A German astronomer named Karl Schwarzschild first proposed what we now call black holes in 1916. While stationed on the front lines in WWI, he solved the equations of Einstein's general relativity in a new and puzzling way. Between calculations of artillery trajectories, Schwarzschild figured out that an enormous amount of mass, like that of a very dense star, concentrated in a small area, would warp the fabric of space-time so severely that nothing, not even light, could escape its gravitational pull.
For decades, physicists were skeptical that Schwarzschild's calculations were anything more than theory. But today satellite telescopes probing deep into space are discovering regions with enormous gravitational pull that most scientists believe are black holes. Schwarzschild's theory now seems to be reality.
So here's the question: if you're trying to figure out what happens in the depths of a black hole, where an entire star is crushed to a tiny speck, do you use general relativity because the star is incredibly heavy or quantum mechanics because it's incredibly tiny?
Well, that's the problem. Since the center of a black hole is both tiny and heavy, you can't avoid using both theories at the same time. And when we try to put the two theories together in the realm of black holes, they conflict. It breaks down. They give nonsensical predictions. And the universe is not nonsensical; it's got to make sense.
EDWARD WITTEN (Institute for Advanced Study): Quantum mechanics works really well for small things, and general relativity works really well for stars and galaxies, but the atoms, the small things, and the galaxies, they're part of the same universe. So there has to be some description that applies to everything. So we can't have one description for atoms and one for stars.
BRIAN GREENE: Now, with string theory, we think we may have found a way to unite our theory of the large and our theory of the small and make sense of the universe at all scales and all places. Instead of a multitude of tiny particles, string theory proclaims that everything in the universe, all forces and all matter is made of one single ingredient, tiny vibrating strands of energy known as strings.
MICHAEL B. GREEN: A string can wiggle in many different ways, whereas, of course, a point can't. And the different ways in which the string wiggles represent the different kinds of elementary particles.
MICHAEL DUFF: It's like a violin string, and it can vibrate just like violin strings can vibrate. Each note if, you like, describes a different particle.
MICHAEL B. GREEN: So it has incredible unification power, it unifies our understanding of all these different kinds of particles.
EDWARD WITTEN: So unity of the different forces and particles is achieved because they all come from different kinds of vibrations of the same basic string.
BRIAN GREENE: It's a simple idea with far-reaching consequences.
JOSEPH LYKKEN (Fermilab): What string theory does is it holds out the promise that, "Look, we can really understand questions that you might not even have thought were scientific questions: questions about how the universe began, why the universe is the way it is at the most fundamental level." The idea that a scientific theory that we already have in our hands could answer the most basic questions is extremely seductive.
BRIAN GREENE: But this seductive new theory is also controversial. Strings, if they exist, are so small, there's little hope of ever seeing one.
JOSEPH LYKKEN: String theory and string theorists do have a real problem. How do you actually test string theory? If you can't test it in the way that we test normal theories, it's not science, it's philosophy, and that's a real problem.
S. JAMES GATES, JR.: If string theory fails to provide a testable prediction, then nobody should believe it. On the other hand, there is a kind of elegance to these things, and given the history of how theoretical physics has evolved thus far, it is totally conceivable that some if not all of these ideas will turn out to be correct.
STEVEN WEINBERG: I think, a hundred years from now, this particular period, when most of the brightest young theoretical physicists worked on string theory, will be remembered as a heroic age when theorists tried and succeeded to develop a unified theory of all the phenomena of nature. On the other hand, it may be remembered as a tragic failure. My guess is that it will be something like the former rather than the latter. But ask me a hundred years from now, then I can tell you.
BRIAN GREENE: Our understanding of the universe has come an enormously long way during the last three centuries. Just consider this. Isaac Newton, who was perhaps the greatest scientist of all time, once said, "I have been like a boy playing on the sea shore, diverting myself in now and then finding a smoother pebble or a prettier shell than usual, while the great ocean of truth lay before me, all undiscovered."
And yet, two hundred and fifty years later, Albert Einstein, who was Newton's true successor, was able to seriously suggest that this vast ocean, all the laws of nature, might be reduced to a few fundamental ideas expressed by a handful of mathematical symbols.
And today, a half century after Einstein's death, we may at last be on the verge of fulfilling his dream of unification with string theory. But where did this daring and strange new theory come from? How does string theory achieve the ultimate unification of the laws of the large and the laws of the small? And how will we know if it's right or wrong?
SHELDON LEE GLASHOW: No experiment can ever check up what's going on at the distances that are being studied. The theory is permanently safe. Is that a theory of physics or a philosophy?
STEVEN WEINBERG: It isn't written in the stars that we're going to succeed, but in the end we hope we will have a single theory that governs everything.
Broadcast Credits
The Elegant Universe: Part 1
- Hosted by
- Brian Greene
- Based on
- The Elegant Universe by Brian Greene
-
Edited by
(Einstein's Dream and String's the Thing) - Jonathan Sahula
-
Edited by
(Welcome to the 11th Dimension) - Dick Bartlett
-
Written, Produced and Directed by
(Einstein's Dream and String's the Thing) - Joseph McMaster
-
Written, Produced and Directed by
(Welcome to the 11th Dimension) -
Julia Cort
Joseph McMaster - Series Producer and Director
- David Hickman
- Series Coordinating Producer
- Andrea Cross
- Series Producer
- Joseph McMaster
- Director of Photography
- Mike Coles
- Sound Recordist
- Keith Rodgerson
- Assistant Camera
- Richard Comrie
- Animation
-
Edgeworx, Inc.
Red Vision -
Visual Effects Supervisors
(Einstein's Dream and String's the Thing) -
John Bair
Jonathan Sahula -
Visual Effects Supervisors
(Welcome to the 11th Dimension) -
John Bair
Dick Bartlett - Music composed by
- Ed Tomney
- Additional Music
- Asche & Spencer
-
Associate Producers
(Einstein's Dream and String's the Thing) -
Barbara Park
Marty Johnson
Jennifer Callahan
Rob Meyer -
Associate Producers
(Welcome to the 11th Dimension) -
Barbara Park
Marty Johnson
Rob Meyer
Julie Crawford
Jennifer Callahan
-
Additional Editing
(Einstein's Dream) -
Dick Bartlett
Rebecca Nieto -
Additional Editing
(String's the Thing) - Rebecca Nieto
-
Additional Editing
(Welcome to the 11th Dimension) -
Jonathan Sahula
Rebecca Nieto -
Additional Camera
(Einstein's Dream and String's the Thing) - Brian Dowley
-
Additional Camera
(Welcome to the 11th Dimension) - James Callanan
-
Studio Art Director
(Einstein's Dream and String's the Thing) - Sabina Sattar
-
Set Builders
(Einstein's Dream and String's the Thing) -
Alistair Bell
Jason Chalmers -
Extras Costume Designer
(Einstein's Dream and String's the Thing) - Agnes Treplin
-
Make-up
(Einstein's Dream and String's the Thing) -
Sarah Berry
Tony Lilley -
Cellist
(Einstein's Dream and String's the Thing) - Kate Sawbridge
-
Cellist
(Welcome to the 11th Dimension) - Nicole Cariglia
-
Production Managers, London
(Einstein's Dream and String's the Thing) -
Jane Moss
Tessa Pemberton -
Production Managers, London
(Welcome to the 11th Dimension) -
Jane Moss
Tessa Pemberton
Catherine Alen-Buckley - Online Editor
- David Tecson
- Colorist
- Greg Dildine, Finish Editorial
-
Audio Mix
(Einstein's Dream and String's the Thing) -
Heart Punch Studio
John Jenkins, WGBH -
Audio Mix
(Welcome to the 11th Dimension) - John Jenkins, WGBH
-
Archival Material
(Einstein's Dream) -
AIP Emilio Segrè Visual Archives
AP / Wide World Photos
BPK, Berlin
California Institute of Technology
Philippe Halsman, Halsman Estate
Image Archive of the ETH-Library, Zurich
Image Bank Film / Archive Films / Getty Images
Library of Congress, Prints & Photographs Division
Lotte Jacobi Collection, University of New Hampshire
Lucien Aigner ©The Lucien Aigner Trust
Manoogian Collection
NASA and Space Telescope Science Institute
National Archives and Records Administration
Pittsburgh Post-Gazette
Science Museum / Science & Society Picture Library
ullstein bild Berlin
Underwood & Underwood / CORBIS -
Archival Material
(String's the Thing) -
BPK, Berlin
Brown Brothers, Sterling, PA
CERN
The New York Times
SCIENCE
Sveriges Television AB -
Archival Material
(Welcome to the 11th Dimension) -
AP / Wide World Photos
Image Bank Film/Archive Films/Getty Images
Science Museum/Science & Society Picture Library
ullstein bild Berlin -
Special Thanks
(Einstein's Dream) -
Promotional Sponsor SEED Magazine
John Charap
The Forrest Family
Andrew J. Hanson
Institute for Advanced Study
Kodak Entertainment Imaging
Light Street, LLC
The Maskin Family
Muse Hotel, New York
Gretchen Sinnett
Georgia Whidden -
Special Thanks
(String's the Thing) -
Promotional Sponsor SEED Magazine
Blue Night Records
The Forrest Family
Andrew J. Hanson
Institute for Advanced Study
Kodak Entertainment Imaging
Light Street, LLC
Muse Hotel, New York
Gretchen Sinnett
Georgia Whidden -
Special Thanks
(Welcome to the 11th Dimension) -
Promotional Sponsor SEED Magazine
The Forrest Family
Institute for Advanced Study
Kodak Entertainment Imaging
Light Street, LLC
Muse Hotel, New York
Georgia Whidden
White Sands Space Harbor
- Advisors
-
Melissa Franklin, Harvard University
Peter Galison, Harvard University
Jim Gates, University of Maryland
Paul Hickman, CESAME, Northeastern University
Joseph Polchinski, University of California, Santa Barbara
Frederick Stein, American Physical Society
Paul Steinhardt, Princeton University
Edwin Taylor, Massachusetts Institute of Technology
Stamatis Vokos, Seattle Pacific University
Steven Weinberg, University of Texas at Austin - NOVA Series Graphics
- National Ministry of Design
- NOVA Theme
-
Mason Daring
Martin Brody
Michael Whalen -
Post Production Online Editors
(Einstein's Dream and String's the Thing) -
Spencer Gentry
Mark Steele -
Post Production Online Editor
(Welcome to the 11th Dimension) - Spencer Gentry
- Closed Captioning
- The Caption Center
- NOVA Administrator
- Queene Coyne
- Publicity
-
Jonathan Renes
Diane Buxton
Tom Stebbins - Senior Researcher
- Ethan Herberman
- Production Coordinator
- Linda Callahan
- Unit Managers
-
Holly Archibald
Lola Norman-Salako - Paralegal
- Nancy Marshall
- Legal Counsel
- Susan Rosen Shishko
- Post Production Assistant
- Patrick Carey
- Associate Producer, Post Production
- Nathan Gunner
- Post Production Supervisor
- Regina O'Toole
- Post Production Editor
- Rebecca Nieto
- Post Production Manager
- Maureen Barden Lynch
- Supervising Producers
-
Lisa D'Angelo
Stephen Sweigart - Producer, Special Projects
- Susanne Simpson
- Coordinating Producer
- Laurie Cahalane
- Senior Science Editor
- Evan Hadingham
- Senior Series Producer
- Melanie Wallace
- Managing Director
- Alan Ritsko
- Senior Executive Producer
- Paula S. Apsell
A NOVA Production for WGBH/Boston and Channel 4 in association with David Hickman Films, Sveriges Television and Norddeutscher Rundfunk
© 2003 WGBH Educational Foundation
All rights reserved
Any opinions, findings, and conclusions or recommendations expressed in this material are those of the author(s) and do not necessarily reflect the views of the National Science Foundation.
- Image credit: (image composite) © WGBH/NOVA
Preview
Notice: Undefined index: preview_video_length in /home/nova/zend/application/templates/resources/multi-ep-pro.phtml on line 497
Full Program | 53:49
Full program available for streaming through ...
Watch Online
Full program available
Soon
The Elegant Universe: Pt 2
String's the Thing: Do miniscule vibrating strands of energy hold the key to a unified theory of physics? Airing July 18, 2012 at 9 pm on PBS Aired July 18, 2012 on PBS
- Originally aired 10.28.03
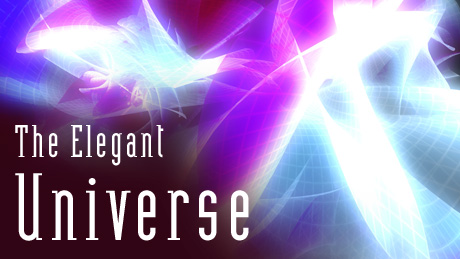
Program Description
Transcript
The Elegant Universe: Part 2
PBS Airdate: October 28, 2003
NARRATOR: Now, on NOVA, take a thrill ride into a world stranger than science fiction, where you play the game by breaking some rules, where a new view of the universe pushes you beyond the limits of your wildest imagination. This is the world of "string theory," a way of describing every force and all matter from an atom to earth, to the end of the galaxies—from the birth of time to its final tick, in a single theory, a "Theory of Everything." Our guide to this brave new world is Brian Greene, the bestselling author and physicist.
BRIAN GREENE (Columbia University): And no matter how many times I come here, I never seem to get used to it.
NARRATOR: Can he help us solve the greatest puzzle of modern physics—that our understanding of the universe is based on two sets of laws that don't agree?
NARRATOR: Resolving that contradiction eluded even Einstein, who made it his final quest. After decades, we may finally be on the verge of a breakthrough. The solution is strings, tiny bits of energy vibrating like the strings on a cello, a cosmic symphony at the heart of all reality. But it comes at a price: parallel universes and 11 dimensions, most of which you've never seen.
BRIAN GREENE: We really may live in a universe with more dimensions than meet the eye.
AMANDA PEET (University of Toronto): People who have said that there were extra dimensions of space have been labeled crackpots, or people who are bananas.
NARRATOR: A mirage of science and mathematics or the ultimate theory of everything?
S. JAMES GATES, JR. (University of Maryland): If string theory fails to provide a testable prediction, then nobody should believe it.
SHELDON LEE GLASHOW: Is that a theory of physics, or a philosophy?
BRIAN GREENE: One thing that is certain is that string theory is already showing us that the universe may be a lot stranger than any of us ever imagined.
NARRATOR: Coming up tonight...
GABRIELE VENEZIANO (CERN): We accidentally discovered string theory.
NARRATOR: ...the humble beginnings of a revolutionary idea.
LEONARD SUSSKIND (Stanford University): I was completely convinced it was going to say, "Susskind is the next Einstein."
JOSEPH LYKKEN (Fermilab): This seemed crazy to people.
LEONARD SUSSKIND: I was depressed, I was unhappy. The result was I went home and got drunk.
NARRATOR: Obsession drives scientists to pursue the Holy Grail of physics, but are they ready for what they discover? Step into the bizarre world of the Elegant Universe right now.
BRIAN GREENE: It's a little known secret but for more than half a century a dark cloud has been looming over modern science. Here's the problem: our understanding of the universe is based on two separate theories. One is Einstein's general theory of relativity—that's a way of understanding the biggest things in the universe, things like stars and galaxies. But the littlest things in the universe, atoms and subatomic particles, play by an entirely different set of rules called, "quantum mechanics."
These two sets of rules are each incredibly accurate in their own domain but whenever we try to combine them, to solve some of the deepest mysteries in the universe, disaster strikes.
Take the beginning of the universe, the "big bang." At that instant a tiny nugget erupted violently. Over the next 14 billion years the universe expanded and cooled into the stars, galaxies and planets we see today. But if we run the cosmic film in reverse, everything that's now rushing apart comes back together, so the universe gets smaller, hotter and denser as we head back to the beginning of time.
As we reach the big bang, when the universe was both enormously heavy and incredibly tiny, our projector jams. Our two laws of physics, when combined, break down.
But what if we could unite quantum mechanics and general relativity and see the cosmic film in its entirety?
Well, a new set of ideas called "string theory" may be able to do that. And if it's right, it would be one of the biggest blockbusters in the history of science. Someday, string theory may be able to explain all of nature, from the tiniest bits of matter to the farthest reaches of the cosmos, using just one single ingredient: tiny vibrating strands of energy called strings.
But why do we have to rewrite the laws of physics to accomplish this? Why does it matter if the two laws that we have are incompatible? Well, you can think of it like this. Imagine you lived in a city ruled not by one set of traffic laws, but by two separate sets of laws that conflicted with each other. As you can see it would be pretty confusing.
To understand this place, you'd need to find a way to put those two conflicting sets of laws together into one all-encompassing set that makes sense.
MICHAEL DUFF (University of Michigan): We work on the assumption that there is a theory out there, and it's our job, if we're sufficiently smart and sufficiently industrious, to figure out what it is.
STEVEN WEINBERG (University of Texas at Austin): We don't have a guarantee—it isn't written in the stars that we're going to succeed—but in the end we hope we will have a single theory that governs everything.
BRIAN GREENE: But before we can find that theory, we need to take a fantastic journey to see why the two sets of laws we have conflict with each other. And the first stop on this strange trip is the realm of very large objects.
To describe the universe on large scales we use one set of laws, Einstein's general theory of relativity, and that's a theory of how gravity works. General relativity pictures space as sort of like a trampoline, a smooth fabric that heavy objects like stars and planets can warp and stretch.
Now, according to the theory, these warps and curves create what we feel as gravity. That is, the gravitational pull that keeps the earth in orbit around the sun is really nothing more than our planet following the curves and contours that the sun creates in the spatial fabric.
But the smooth, gently curving image of space predicted by the laws of general relativity is not the whole story. To understand the universe on extremely small scales, we have to use our other set of laws, quantum mechanics. And as we'll see, quantum mechanics paints a picture of space so drastically different from general relativity that you'd think they were describing two completely separate universes.
To see the conflict between general relativity and quantum mechanics we need to shrink way, way, way down in size. And as we leave the world of large objects behind and approach the microscopic realm, the familiar picture of space in which everything behaves predictably begins to be replaced by a world with a structure that is far less certain.
And if we keep shrinking, getting billions and billion of times smaller than even the tiniest bits of matter—atoms and the tiny particles inside of them—the laws of the very small, quantum mechanics, say that the fabric of space becomes bumpy and chaotic. Eventually we reach a world so turbulent that it defies common sense.
Down here, space and time are so twisted and distorted that the conventional ideas of left and right, up and down, even before and after, break down. There's no way to tell for certain that I'm here, or here or both places at once. Or maybe I arrived here before I arrived here.
In the quantum world you just can't pin everything down. It's an inherently wild and frenetic place.
WALTER H.G. LEWIN (Massachusetts Institute of Technology): The laws in the quantum world are very different from the laws that we are used to. And is that surprising? Why should the world of the very small, at an atomic level, why should that world obey the same kind of rules and laws that we are used to in our world, with apples and oranges and walking around on the street? Why would that world behave the same way?
BRIAN GREENE: The fluctuating jittery picture of space and time predicted by quantum mechanics is in direct conflict with the smooth, orderly, geometric model of space and time described by general relativity. But we think that everything, from the frantic dance of subatomic particles to the majestic swirl of galaxies, should be explained by just one grand physical principle, one master equation.
If we can find that equation, how the universe really works at every time and place will at last be revealed. You see, what we need is a theory that can cope with the very tiny and the very massive, one that embraces both quantum mechanics and general relativity, and never breaks down, ever.
For physicists, finding a theory that unites general relativity and quantum mechanics is the Holy Grail, because that framework would give us a single mathematical theory that describes all the forces that rule our universe. General relativity describes the most familiar of those forces: gravity. But quantum mechanics describes three other forces: the strong nuclear force that's responsible for gluing protons and neutrons together inside of atoms; electromagnetism, which produces light, electricity and magnetic attraction; and the weak nuclear force: that's the force responsible for radioactive decay.
Albert Einstein spent the last 30 years of his life searching for a way to describe the forces of nature in a single theory, and now string theory may fulfill his dream of unification.
For centuries, scientists have pictured the fundamental ingredients of nature—atoms and the smaller particles inside of them—as tiny balls or points. But string theory proclaims that at the heart of every bit of matter is a tiny, vibrating strand of energy called a string. And a new breed of scientist believes these miniscule strings are the key to uniting the world of the large and the world of the small in a single theory.
JOSEPH LYKKEN: The idea that a scientific theory that we already have in our hands could answer the most basic questions is extremely seductive.
S. JAMES GATES, JR.: For about 2,000 years, all of our physics essentially has been based on...essentially we were talking about billiard balls. The very idea of the string is such a paradigm shift, because instead of billiard balls, you have to use little strands of spaghetti.
BRIAN GREENE: But not everyone is enamored of this new theory. So far no experiment has been devised that can prove these tiny strings exist.
SHELDON LEE GLASHOW (Boston University): And let me put it bluntly. There are physicists and there are string theorists. It is a new discipline, a new—you may call it a tumor—you can call it what you will, but they have focused on questions which experiment cannot address. They will deny that, these string theorists, but it's a kind of physics which is not yet testable, it does not make predictions that have anything to do with experiments that can be done in the laboratory or with observations that could be made in space or from telescopes. And I was brought up to believe, and I still believe, that physics is an experimental science. It deals with the results to experiments, or in the case of astronomy, observations.
BRIAN GREENE: From the start, many scientists thought string theory was simply too far out. And frankly, the strange way the theory evolved—in a series of twists, turns and accidents—only made it seem more unlikely.
In the late 1960s a young Italian physicist, named Gabriele Veneziano, was searching for a set of equations that would explain the strong nuclear force, the extremely powerful glue that holds the nucleus of every atom together binding protons to neutrons. As the story goes, he happened on a dusty book on the history of mathematics, and in it he found a 200-year old equation, first written down by a Swiss mathematician, Leonhard Euler. Veneziano was amazed to discover that Euler's equations, long thought to be nothing more than a mathematical curiosity, seemed to describe the strong force.
He quickly published a paper and was famous ever after for this "accidental" discovery.
GABRIELE VENEZIANO (CERN): I see occasionally, written in books, that, uh, that this model was invented by chance or was, uh, found in the math book, and, uh, this makes me feel pretty bad. What is true is that the function was the outcome of a long year of work, and we accidentally discovered string theory.
BRIAN GREENE: However it was discovered, Euler's equation, which miraculously explained the strong force, took on a life of its own. This was the birth of string theory. Passed from colleague to colleague, Euler's equation ended up on the chalkboard in front of a young American physicist, Leonard Susskind.
LEONARD SUSSKIND: To this day I remember the formula. The formula was... and I looked at it, and I said, "This is so simple even I can figure out what this is."
BRIAN GREENE: Susskind retreated to his attic to investigate. He understood that this ancient formula described the strong force mathematically, but beneath the abstract symbols he had caught a glimpse of something new.
LEONARD SUSSKIND: And I fiddled with it, I monkeyed with it. I sat in my attic, I think for two months on and off. But the first thing I could see in it, it was describing some kind of particles which had internal structure which could vibrate, which could do things, which wasn't just a point particle. And I began to realize that what was being described here was a string, an elastic string, like a rubber band, or like a rubber band cut in half. And this rubber band could not only stretch and contract, but wiggle. And marvel of marvels, it exactly agreed with this formula.
I was pretty sure at that time that I was the only one in the world who knew this.
BRIAN GREENE: Susskind wrote up his discovery introducing the revolutionary idea of strings. But before his paper could be published it had to be reviewed by a panel of experts.
LEONARD SUSSKIND: I was completely convinced that when it came back it was going to say, "Susskind is the next Einstein," or maybe even, "the next Newton." And it came back saying, "this paper's not very good, probably shouldn't be published."
I was truly knocked off my chair. I was depressed, I was unhappy. I was saddened by it. It made me a nervous wreck, and the result was I went home and got drunk.
BRIAN GREENE: As Susskind drowned his sorrows over the rejection of his far out idea, it appeared string theory was dead.
Meanwhile, mainstream science was embracing particles as points, not strings. For decades, physicists had been exploring the behavior of microscopic particles by smashing them together at high speeds and studying those collisions. In the showers of particles produced, they were discovering that nature is far richer than they thought.
SHELDON LEE GLASHOW: Once a month there'd be a discovery of a new particle: the Rho meson, the Omega particle, the B particle, the B1 particle, the B2 particle, Phi, Omega...more letters were used than exist in most alphabets. It was a population explosion of particles.
STEVEN WEINBERG: It was a time when graduate students would run through the halls of a physics building saying they discovered another particle, and it fit the theories. And it was all so exciting.
BRIAN GREENE: And in this zoo of new particles, scientists weren't just discovering building blocks of matter. Leaving string theory in the dust, physicists made a startling and strange prediction: that the forces of nature can also be explained by particles.
Now, this is a really weird idea, but it's kind of like a game of catch in which the players like me and me are particles of matter. And the ball we're throwing back and forth is a particle of force. It's called a messenger particle.
For example, in the case of magnetism, the electromagnetic force—this ball—would be a photon. The more of these messenger particles or photons that are exchanged between us, the stronger the magnetic attraction. And scientists predicted that it's this exchange of messenger particles that creates what we feel as force. Experiments confirmed these predictions with the discovery of the messenger particles for electromagnetism, the strong force and the weak force.
And using these newly discovered particles scientists were closing in on Einstein's dream of unifying the forces. Particle physicists reasoned that if we rewind the cosmic film to the moments just after the big bang, some 14 billion years ago when the universe was trillions of degrees hotter, the messenger particles for electromagnetism and the weak force would have been indistinguishable. Just as cubes of ice melt into water in the hot sun, experiments show that as we rewind to the extremely hot conditions of the Big Bang, the weak and electromagnetic forces meld together and unite into a single force called "the electroweak."
And physicists believe that if you roll the cosmic film back even further, the electroweak would unite with the strong force in one grand "super-force." Although that has yet to be proven, quantum mechanics was able to explain how three of the forces operate on the subatomic level.
SHELDON LEE GLASHOW: And all of a sudden we had a consistent theory of elementary particle physics, which allows us to describe all of the interactions—weak, strong and electromagnetic—in the same language. It all made sense, and it's all in the textbooks.
STEVEN WEINBERG: Everything was converging toward a simple picture of the known particles and forces, a picture which eventually became known as the "Standard Model." I think I gave it that name.
BRIAN GREENE: The inventors of the Standard Model, both the name and the theory, were the toasts of the scientific community, receiving Nobel Prize after Nobel Prize. But behind the fanfare was a glaring omission. Although the standard model explained three of the forces that rule the world of the very small, it did not include the most familiar force, gravity.
Overshadowed by the Standard Model, string theory became a backwater of physics.
GABRIELE VENEZIANO: Most people in our community lost, completely, interest in string theory. They said, "Okay, that was a very nice elegant thing but had nothing to do with nature."
S. JAMES GATES, JR.: It's not taken seriously by much of the community, but the early pioneers of string theory are convinced that they can smell reality and continue to pursue the idea.
BRIAN GREENE: But the more these diehards delved into string theory the more problems they found.
JOSEPH LYKKEN: Early string theory had a number of problems. One was that it predicted a particle which we know is unphysical. It's what's called a "tachyon," a particle that travels faster than light.
JOHN H. SCHWARZ (California Institute of Technology): There was this discovery that the theory requires ten dimensions, which is very disturbing, of course, since it's obvious that that's more than there are.
CUMRUN VAFA (Harvard University): It had this massless particle which was not seen in experiments.
MICHAEL B. GREEN: So these theories didn't seem to make sense.
JOSEPH LYKKEN: This seemed crazy to people.
CUMRUN VAFA: Basically, string theory was not getting off the ground.
JOSEPH LYKKEN: People threw up their hands and said, "This can't be right."
BRIAN GREENE: By 1973, only a few young physicists were still wrestling with the obscure equations of string theory. One was John Schwarz, who was busy tackling string theory's numerous problems, among them a mysterious massless particle predicted by the theory but never seen in nature, and an assortment of anomalies or mathematical inconsistencies.
JOHN H. SCHWARZ: We spent a long time trying to fiddle with the theory. We tried all sorts of ways of making the dimension be four, getting rid of these massless particles and the tachyons and so on, but it was always ugly and unconvincing.
BRIAN GREENE: For four years, Schwarz tried to tame the unruly equations of string theory, changing, adjusting, combining and recombining them in different ways. But nothing worked. On the verge of abandoning string theory, Schwarz had a brainstorm: perhaps his equations were describing gravity. But that meant reconsidering the size of these tiny strands of energy.
JOHN H. SCHWARZ: We weren't thinking about gravity up 'til that point. But as soon as we suggested that maybe we should be dealing with a theory of gravity, we had to radically change our view of how big these strings were.
BRIAN GREENE: By supposing that strings were a hundred billion billion times smaller than an atom, one of the theory's vices became a virtue.
The mysterious particle John Schwarz had been trying to get rid of now appeared to be a graviton, the long sought after particle believed to transmit gravity at the quantum level.
String theory had produced the piece of the puzzle missing from the standard model. Schwarz submitted for publication his groundbreaking new theory describing how gravity works in the subatomic world.
JOHN H. SCHWARZ: It seemed very obvious to us that it was right. But there was really no reaction in the community whatsoever.
BRIAN GREENE: Once again string theory fell on deaf ears. But Schwarz would not be deterred. He had glimpsed the Holy Grail. If strings described gravity at the quantum level, they must be the key to unifying the four forces. He was joined in this quest by one of the only other scientists willing to risk his career on strings, Michael Green.
MICHAEL B. GREEN (University of Cambridge): In a sense, I think, we had a quiet confidence that the string theory was obviously correct, and it didn't matter much if people didn't see it at that point. They would see it down the line.
BRIAN GREENE: But for Green's confidence to pay off, he and Schwarz would have to confront the fact that in the early 1980s, string theory still had fatal flaws in the math known as "anomalies." An anomaly is just what it sounds like. It's something that's strange or out of place, something that doesn't belong.
Now this kind of anomaly is just weird. But mathematical anomalies can spell doom for a theory of physics. They're a little complicated, so here's a simple example: let's say we have a theory in which these two equations describe one physical property of our universe. Now if I solve this equation over here, and I find x=1, and if I solve this equation over here and find x=2, I know my theory has anomalies because there should only be one value for X. Unless I can revise my equations to get the same value for X on both sides, the theory is dead.
In the early 1980s, string theory was riddled with mathematical anomalies kind of like these, although the equations were much more complex. The future of the theory depended on ridding the equations of these fatal inconsistencies.
After Schwarz and Green battled the anomalies in string theory for five years, their work culminated late one night in the summer of 1984.
JOHN H. SCHWARZ: It was widely believed that these theories must be inconsistent because of anomalies. Well, for no really good reason, I just felt that had to be wrong because I, I felt, "String theory has got to be right, therefore there can't be anomalies." So we decided, "We've got to calculate these things."
BRIAN GREENE: Amazingly it all boiled down to a single calculation. On one side of the blackboard they got 496. And if they got the matching number on the other side it would prove string theory was free of anomalies.
MICHAEL B. GREEN: I do remember a particular moment, when John Schwarz and I were talking at the blackboard and working out these numbers which had to fit, and they just had to match exactly. I remember joking with John Schwarz at that moment, because there was thunder and lightning—there was a big mountain storm in Aspen at that moment—and I remember saying something like, you know, "We must be getting pretty close, because the gods are trying to prevent us completing this calculation." And, indeed, they did match.
BRIAN GREENE: The matching numbers meant the theory was free of anomalies. And it had the mathematical depth to encompass all four forces.
JOHN H. SCHWARZ: So we recognized not only that the strings could describe gravity but they could also describe the other forces. So we spoke in terms of unification. And we saw this as a possibility of realizing the dream that Einstein had expressed in his later years, of unifying the different forces in some deeper framework.
MICHAEL B. GREEN: We felt great. That was an extraordinary moment, because we realized that no other theory had ever succeeded in doing that.
JOHN H. SCHWARZ: But by now, it's like crying wolf. Each time we had done something, I figured everyone's going to be excited, and they weren't. So I, I figured...by now I didn't expect much of a reaction.
BRIAN GREENE: But this time the reaction was explosive. In less than a year, the number of string theorists leapt from just a handful to hundreds.
MICHAEL B. GREEN: Up to that moment, the longest talk I'd ever given on the subject was five minutes at some minor conference. And then, suddenly, I was invited all over the world to give talks and lectures and so forth.
BRIAN GREENE: String theory was christened "The Theory of Everything."
In early fall of 1984, I came here, to Oxford University, to begin my graduate studies in physics. Some weeks after, I saw a poster for a lecture by Michael Green. I didn't know who he was, but, then again, I really didn't know who anybody was. But the title of the lecture was something like "The Theory of Everything." So how could I resist?
This elegant new version of string theory seemed capable of describing all the building blocks of nature.
Here's how: inside every grain of sand are billions of tiny atoms. Every atom is made of smaller bits of matter, electrons orbiting a nucleus made of protons and neutrons, which are made of even smaller bits of matter called quarks. But string theory says this is not the end of the line. It makes the astounding claim that the particles making up everything in the universe are made of even smaller ingredients, tiny wiggling strands of energy that look like strings. Each of these strings is unimaginably small. In fact, if an atom were enlarged to the size of the solar system, a string would only be as large as a tree!
And here's the key idea. Just as different vibrational patterns or frequencies of a single cello string create what we hear as different musical notes, the different ways that strings vibrate give particles their unique properties, such as mass and charge. For example, the only difference between the particles making up you and me and the particles that transmit gravity and the other forces is the way these tiny strings vibrate.
Composed of an enormous number of these oscillating strings, the universe can be thought of as a grand cosmic symphony. And this elegant idea resolves the conflict between our jittery unpredictable picture of space on the subatomic scale and our smooth picture of space on the large scale.
It's the jitteriness of quantum theory versus the gentleness of Einstein's general theory of relativity that makes it so hard to bridge the two, to stitch them together. Now, what string theory does, it comes along and basically calms the jitters of quantum mechanics. It spreads them out by virtue of taking the old idea of a point particle and spreading it out into a string. So the jittery behavior is there, but it's just sufficiently less violent that quantum theory and general relativity stitch together perfectly within this framework.
It's a triumph of mathematics. With nothing but these tiny vibrating strands of energy, string theorists claim to be fulfilling Einstein's dream of uniting all forces and all matter.
But this radical new theory contains a chink in its armor.
SHELDON LEE GLASHOW: No experiment can ever check up what's going on at the distances that are being studied. No observation can relate to these tiny distances or high energies. That is to say, there ain't no experiment that could be done, nor is there any observation that could be made, that would say, "You guys are wrong." The theory is safe, permanently safe. Is that a theory of physics or a philosophy? I ask you.
MICHAEL B. GREEN: People often criticize string theory for saying that it's very far removed from any direct experimental test, and it's...surely it's not really, um, um, a branch of physics, for that reason. And I, my response to that is simply that they're going to be proved wrong.
BRIAN GREENE: Making string theory even harder to prove, is that, in order to work, the complex equations require something that sounds like it's straight out of science fiction: extra dimensions of space.
AMANDA PEET: We've always thought, for centuries, that there was only what we can see. You know, this dimension, that one, and another one. There was only three dimensions of space and one of time. And people who've said that there were extra dimensions of space have been labeled as, you know, crackpots, or people who were bananas. Well, string theory really predicts it.
BRIAN GREENE: To be taken seriously, string theorists had to explain how this bizarre prediction could be true. And they claim that the far out idea of extra dimensions may be more down to earth than you'd think.
Let me show you what I mean. I'm off to see a guy who was one of the first people to think about this strange idea. I'm supposed to meet him at four o'clock at his apartment at Fifth Avenue and 93rd Street, on the second floor. Now, in order to get to this meeting, I need four pieces of information: one for each of the three dimensions of space—a street, an avenue and a floor number—and one more for time, the fourth dimension. You can think about these as the four dimensions of common experience: left-right, back-forth, up-down and time.
As it turns out, the strange idea that there are additional dimensions stretches back almost a century. Our sense that we live in a universe of three spatial dimensions really seems beyond question. But in 1919, Theodor Kaluza, a virtually unknown German mathematician, had the courage to challenge the obvious. He suggested that maybe, just maybe, our universe has one more dimension that for some reason we just can't see.
THEODOR KALUZA (ACTOR): Look. He says here, "I like your idea." So why does he delay?
BRIAN GREENE: You see, Kaluza had sent his idea about an additional spatial dimension to Albert Einstein. And although Einstein was initially enthusiastic, he then seemed to waver, and for two years held up publication of Kaluza's paper. Eventually, Kaluza's paper was published—after Einstein decided extra dimensions were his cup of tea.
Here's the idea. In 1916, Einstein showed that gravity is nothing but warps and ripples in the four familiar dimensions of space and time. Just three years later, Kaluza proposed that electromagnetism might also be ripples. But for that to be true, Kaluza needed a place for those ripples to occur. So Kaluza proposed an additional hidden dimension of space.
But if Kaluza was right, where is this extra dimension? And what would extra dimensions look like? Can we even begin to imagine them? Well, building upon Kaluza's work, the Swedish physicist Oskar Klein suggested an unusual answer.
Take a look at the cables supporting that traffic light. From this far away I can't see that they have any thickness. Each one looks like a line—something with only a single dimension.
But suppose we could explore one of these cables way up close, like from the point of view of an ant. Now a second dimension which wraps around the cable becomes visible. From its point of view, the ant can move forwards and backwards, and it can also move clockwise and counterclockwise.
So dimensions can come in two varieties. They can be long and unfurled like the length of the cable, but they can also be tiny and curled up like the circular direction that wraps around it.
Kaluza and Klein made the wild suggestion that the fabric of our universe might be kind of like the surface of the cable, having both big extended dimensions, the three that we know about, but also tiny, curled up dimensions, curled up so tiny—billions of times smaller than even a single atom—that we just can't see them. And so our perception that we live in a universe with three spatial dimensions may not be correct after all. We really may live in a universe with more dimensions than meet the eye.
So what would these extra dimensions look like? Kaluza and Klein proposed that if we could shrink down billions of times, we'd find one extra tiny, curled up dimension located at every point in space. And just the way an ant can explore the circular dimension that wraps around a traffic light cable, in theory an ant that is billions of times smaller could also explore this tiny, curled up, circular dimension.
This idea that extra dimensions exist all around us lies at the heart of string theory. In fact the mathematics of string theory demand not one, but six extra dimensions, twisted and curled into complex little shapes that might look something like this.
MICHAEL DUFF: If string theory is right we would have to admit that there are really more dimensions out there, and I find that completely mind-blowing.
EDWARD WITTEN (Institute for Advanced Study): If I take the theory as we have it now, literally, I would conclude that the extra dimensions really exist. They're part of nature.
JOSEPH LYKKEN: When we talk about extra dimensions we literally mean extra dimensions of space that are the same as the dimensions of space that we see around us. And the only difference between them has to do with their shape.
BRIAN GREENE: But how could these tiny extra dimensions, curled up into such peculiar shapes, have any effect on our everyday world? Well, according to string theory, shape is everything.
Because of its shape, a French horn can produce dozens of different notes. When you press one of the keys you change the note, because you change the shape of the space inside the horn where the air resonates. And we think the curled up spatial dimensions in string theory work in a similar way.
If we could shrink down small enough to fly into one of these tiny six-dimensional shapes predicted by string theory we would see how the extra dimensions are twisted and curled back on each other, influencing how strings, the fundamental ingredients of our universe, move and vibrate. And this could be the key to solving one of nature's most profound mysteries.
You see, our universe is kind of like a finely tuned machine. Scientists have found that there are about 20 numbers, 20 fundamental constants of nature that give the universe the characteristics we see today. These are numbers like how much an electron weighs, the strength of gravity, the electromagnetic force and the strong and weak forces. Now, as long as we set the dials on our universe machine to precisely the right values for each of these 20 numbers, the machine produces the universe we know and love.
But if we change the numbers by adjusting the settings on this machine even a little bit... the consequences are dramatic.
For example, if I increase the strength of the electromagnetic force, atoms repel one other more strongly, so the nuclear furnaces that make stars shine break down. The stars, including our sun, fizzle out, and the universe as we know it disappears.
So what exactly, in nature, sets the values of these 20 constants so precisely? Well the answer could be the extra dimensions in string theory. That is, the tiny, curled up, six-dimensional shapes predicted by the theory cause one string to vibrate in precisely the right way to produce what we see as a photon and another string to vibrate in a different way producing an electron. So according to string theory, these miniscule extra-dimensional shapes really may determine all the constants of nature, keeping the cosmic symphony of strings in tune.
By the mid 1980s, string theory looked unstoppable, but behind the scenes the theory was in tangles. Over the years, string theorists had been so successful that they had constructed not one, but five different versions of the theory. Each was built on strings and extra dimensions, but in detail, the five theories were not in harmony. In some versions, strings were open-ended strands. In others they were closed loops. At first glance, a couple of versions even required 26 dimensions. All five versions appeared equally valid, but which one was describing our universe?
This was kind of an embarrassment for string theorists because on the one hand, we wanted to say that this might be it, the final description of the universe. But then, in the next breath we had to say, "And it comes in five flavors, five variations." Now there's one universe you expect there to be one theory and not five. So this is an example where more is definitely less.
MICHAEL B GREEN: One attitude that people who didn't like string theory could take was, "Well, you have five theories, so it's not unique."
JOHN H. SCHWARZ: This was a peculiar state of affairs, because we were looking just to describe one theory of nature and not five.
JOSEPH LYKKEN: If there's five of them, well maybe there's smart enough people would find twenty of them. Or maybe there's an infinite number of them, and you're back to just searching around at random for theories of the world.
CUMRUN VAFA: Maybe one of these five string theories is describing our universe—on the other hand, which one? And why? What are the other ones good for?
EDWARD WITTEN: Having five string theories, even though it's big progress, raises the obvious question: if one of those theories describes our universe then who lives in the other four worlds?
BRIAN GREENE: String theory seemed to be losing steam once again. And frustrated by a lack of progress, many physicists abandoned the field.
NARRATOR: Will string theory prove to be a "Theory of Everything" or will it unravel into a "Theory of Nothing?"
Broadcast Credits
The Elegant Universe: Part 2
- Hosted by
- Brian Greene
- Based on
- The Elegant Universe by Brian Greene
-
Edited by
(Einstein's Dream and String's the Thing) - Jonathan Sahula
-
Edited by
(Welcome to the 11th Dimension) - Dick Bartlett
-
Written, Produced and Directed by
(Einstein's Dream and String's the Thing) - Joseph McMaster
-
Written, Produced and Directed by
(Welcome to the 11th Dimension) -
Julia Cort
Joseph McMaster - Series Producer and Director
- David Hickman
- Series Coordinating Producer
- Andrea Cross
- Series Producer
- Joseph McMaster
- Director of Photography
- Mike Coles
- Sound Recordist
- Keith Rodgerson
- Assistant Camera
- Richard Comrie
- Animation
-
Edgeworx, Inc.
Red Vision -
Visual Effects Supervisors
(Einstein's Dream and String's the Thing) -
John Bair
Jonathan Sahula -
Visual Effects Supervisors
(Welcome to the 11th Dimension) -
John Bair
Dick Bartlett - Music composed by
- Ed Tomney
- Additional Music
- Asche & Spencer
-
Associate Producers
(Einstein's Dream and String's the Thing) -
Barbara Park
Marty Johnson
Jennifer Callahan
Rob Meyer -
Associate Producers
(Welcome to the 11th Dimension) -
Barbara Park
Marty Johnson
Rob Meyer
Julie Crawford
Jennifer Callahan
-
Additional Editing
(Einstein's Dream) -
Dick Bartlett
Rebecca Nieto -
Additional Editing
(String's the Thing) - Rebecca Nieto
-
Additional Editing
(Welcome to the 11th Dimension) -
Jonathan Sahula
Rebecca Nieto -
Additional Camera
(Einstein's Dream and String's the Thing) - Brian Dowley
-
Additional Camera
(Welcome to the 11th Dimension) - James Callanan
-
Studio Art Director
(Einstein's Dream and String's the Thing) - Sabina Sattar
-
Set Builders
(Einstein's Dream and String's the Thing) -
Alistair Bell
Jason Chalmers -
Extras Costume Designer
(Einstein's Dream and String's the Thing) - Agnes Treplin
-
Make-up
(Einstein's Dream and String's the Thing) -
Sarah Berry
Tony Lilley -
Cellist
(Einstein's Dream and String's the Thing) - Kate Sawbridge
-
Cellist
(Welcome to the 11th Dimension) - Nicole Cariglia
-
Production Managers, London
(Einstein's Dream and String's the Thing) -
Jane Moss
Tessa Pemberton -
Production Managers, London
(Welcome to the 11th Dimension) -
Jane Moss
Tessa Pemberton
Catherine Alen-Buckley - Online Editor
- David Tecson
- Colorist
- Greg Dildine, Finish Editorial
-
Audio Mix
(Einstein's Dream and String's the Thing) -
Heart Punch Studio
John Jenkins, WGBH -
Audio Mix
(Welcome to the 11th Dimension) - John Jenkins, WGBH
-
Archival Material
(Einstein's Dream) -
AIP Emilio Segrè Visual Archives
AP / Wide World Photos
BPK, Berlin
California Institute of Technology
Philippe Halsman, Halsman Estate
Image Archive of the ETH-Library, Zurich
Image Bank Film / Archive Films / Getty Images
Library of Congress, Prints & Photographs Division
Lotte Jacobi Collection, University of New Hampshire
Lucien Aigner ©The Lucien Aigner Trust
Manoogian Collection
NASA and Space Telescope Science Institute
National Archives and Records Administration
Pittsburgh Post-Gazette
Science Museum / Science & Society Picture Library
ullstein bild Berlin
Underwood & Underwood / CORBIS -
Archival Material
(String's the Thing) -
BPK, Berlin
Brown Brothers, Sterling, PA
CERN
The New York Times
SCIENCE
Sveriges Television AB -
Archival Material
(Welcome to the 11th Dimension) -
AP / Wide World Photos
Image Bank Film/Archive Films/Getty Images
Science Museum/Science & Society Picture Library
ullstein bild Berlin -
Special Thanks
(Einstein's Dream) -
Promotional Sponsor SEED Magazine
John Charap
The Forrest Family
Andrew J. Hanson
Institute for Advanced Study
Kodak Entertainment Imaging
Light Street, LLC
The Maskin Family
Muse Hotel, New York
Gretchen Sinnett
Georgia Whidden -
Special Thanks
(String's the Thing) -
Promotional Sponsor SEED Magazine
Blue Night Records
The Forrest Family
Andrew J. Hanson
Institute for Advanced Study
Kodak Entertainment Imaging
Light Street, LLC
Muse Hotel, New York
Gretchen Sinnett
Georgia Whidden -
Special Thanks
(Welcome to the 11th Dimension) -
Promotional Sponsor SEED Magazine
The Forrest Family
Institute for Advanced Study
Kodak Entertainment Imaging
Light Street, LLC
Muse Hotel, New York
Georgia Whidden
White Sands Space Harbor
- Advisors
-
Melissa Franklin, Harvard University
Peter Galison, Harvard University
Jim Gates, University of Maryland
Paul Hickman, CESAME, Northeastern University
Joseph Polchinski, University of California, Santa Barbara
Frederick Stein, American Physical Society
Paul Steinhardt, Princeton University
Edwin Taylor, Massachusetts Institute of Technology
Stamatis Vokos, Seattle Pacific University
Steven Weinberg, University of Texas at Austin - NOVA Series Graphics
- National Ministry of Design
- NOVA Theme
-
Mason Daring
Martin Brody
Michael Whalen -
Post Production Online Editors
(Einstein's Dream and String's the Thing) -
Spencer Gentry
Mark Steele -
Post Production Online Editor
(Welcome to the 11th Dimension) - Spencer Gentry
- Closed Captioning
- The Caption Center
- NOVA Administrator
- Queene Coyne
- Publicity
-
Jonathan Renes
Diane Buxton
Tom Stebbins - Senior Researcher
- Ethan Herberman
- Production Coordinator
- Linda Callahan
- Unit Managers
-
Holly Archibald
Lola Norman-Salako - Paralegal
- Nancy Marshall
- Legal Counsel
- Susan Rosen Shishko
- Post Production Assistant
- Patrick Carey
- Associate Producer, Post Production
- Nathan Gunner
- Post Production Supervisor
- Regina O'Toole
- Post Production Editor
- Rebecca Nieto
- Post Production Manager
- Maureen Barden Lynch
- Supervising Producers
-
Lisa D'Angelo
Stephen Sweigart - Producer, Special Projects
- Susanne Simpson
- Coordinating Producer
- Laurie Cahalane
- Senior Science Editor
- Evan Hadingham
- Senior Series Producer
- Melanie Wallace
- Managing Director
- Alan Ritsko
- Senior Executive Producer
- Paula S. Apsell
A NOVA Production for WGBH/Boston and Channel 4 in association with David Hickman Films, Sveriges Television and Norddeutscher Rundfunk
© 2003 WGBH Educational Foundation
All rights reserved
Any opinions, findings, and conclusions or recommendations expressed in this material are those of the author(s) and do not necessarily reflect the views of the National Science Foundation.
- Image credit: (image composite) © WGBH
Preview
Notice: Undefined index: preview_video_length in /home/nova/zend/application/templates/resources/multi-ep-pro.phtml on line 497
Full Program | 52:48
Full program available for streaming through ...
Watch Online
Full program available
Soon
The Elegant Universe: Pt 3
Welcome to the 11th Dimension: Will experimental particle physics confirm the wild predictions of string theory? Airing July 25, 2012 at 9 pm on PBS Aired July 25, 2012 on PBS
- Originally aired 11.04.03
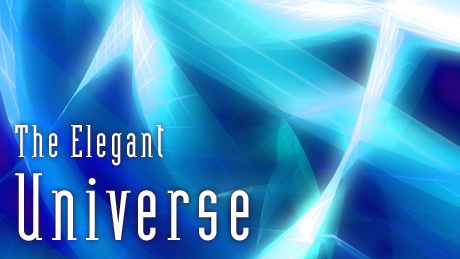
Program Description
Transcript
The Elegant Universe: Part 3
PBS Airdate: November 4, 2003
NARRATOR: Now, on NOVA, take a thrill ride into a world stranger than science fiction, where you play the game by breaking some rules, where a new view of the universe pushes you beyond the limits of your wildest imagination. This is the world of "string theory," a way of describing every force and all matter from an atom to earth, to the end of the galaxies—from the birth of time to its final tick, in a single theory, a "Theory of Everything." Our guide to this brave new world is Brian Greene, the bestselling author and physicist.
BRIAN GREENE (Columbia University): And no matter how many times I come here, I never seem to get used to it.
NARRATOR: Can he help us solve the greatest puzzle of modern physics—that our understanding of the universe is based on two sets of laws that don't agree?
NARRATOR: Resolving that contradiction eluded even Einstein, who made it his final quest. After decades, we may finally be on the verge of a breakthrough. The solution is strings, tiny bits of energy vibrating like the strings on a cello, a cosmic symphony at the heart of all reality. But it comes at a price: parallel universes and 11 dimensions, most of which you've never seen.
BRIAN GREENE: We really may live in a universe with more dimensions than meet the eye.
AMANDA PEET (University of Toronto): People who have said that there were extra dimensions of space have been labeled crackpots, or people who are bananas.
NARRATOR: A mirage of science and mathematics or the ultimate theory of everything?
S. JAMES GATES, JR. (University of Maryland): If string theory fails to provide a testable prediction, then nobody should believe it.
SHELDON LEE GLASHOW (Boston University): Is that a theory of physics, or a philosophy?
BRIAN GREENE: One thing that is certain is that string theory is already showing us that the universe may be a lot stranger than any of us ever imagined.
NARRATOR: Coming up tonight, the undeniable pull of strings.
BRIAN GREENE:The atmosphere was electric. String theory goes through a revolution of its own...
MICHAEL DUFF (University of Michigan): Five different string theories...
BRIAN GREENE: ...and reveals the new shape of things to come.
SAVAS DIMOPOULOS (Stanford University): Perhaps we live on a three-dimensional membrane.
BRIAN GREENE: Our universe might be like a slice of bread.
BRIAN GREENE: We're trapped on just a tiny slice of the higher dimensional universe.
ALAN GUTH (Massachusetts Institute of Technology): That's actually a problem.
NARRATOR: Watch the Elegant Universe right now.
Major funding for NOVA is provided by the Park Foundation.
We see 400 employees in three years. At Microsoft, your potential inspires us to create software that helps you reach it. Your potential, our passion.
Science: it's given us the framework to help make wireless communications clear. Sprint is proud to support NOVA.
Funding for the Elegant Universe is provided by the Alfred P. Sloan Foundation, to enhance public understanding of science and technology.
And by the National Science Foundation, America's investment in the future.
Additional funding is provided by Volkswagen of America.
And by the George D. Smith Fund, and the U.S. Department of Energy, fostering science and security.
Major funding for NOVA is also provided by the Corporation for Public Broadcasting, and viewers like you. Thank you.
BRIAN GREENE: Imagine that we were able to control space or control time. The kinds of things that we'd be able to do would be amazing. I might be able to go from here...to here...to here...to here...and over to here in only an instant.
Now, we all think that this kind of trip would be impossible. And it probably is. But in the last few years, our ideas about the true nature of space and time have been going through some changes. And things that used to seem like science fiction are looking not-so-far-fetched.
It's all thanks to a revolution in physics called "string theory," which is offering a whole new perspective on the inner workings of the universe.
JOSEPH LYKKEN (Fermilab): String theory holds out the promise that we can really understand questions of why the universe is the way it is at the most fundamental level.
DAVID GROSS (University of California, Santa Barbara): String theory is really the Wild West of physics.
MICHAEL B. GREEN (University of Cambridge): This is an area of theoretical physics which is so radically different from anything that's been before.
BRIAN GREENE: This radical new theory starts with a simple premise: that everything in the universe, the Earth, these buildings, even forces like gravity and electricity, are made up of incredibly tiny, vibrating strands of energy called "strings."
And small as they are, strings are changing everything we thought we knew about the universe, especially our ideas about the nature of space.
To see how, let's first shrink all of space to a more manageable size. Imagine that the whole universe consisted of nothing more than my hometown, Manhattan. So now, just one borough of New York City makes up the entire fabric of space.
And just for kicks, let's also imagine that I'm the CEO of a large corporation with offices on Wall Street. And because time is money, I need to find the quickest route from my apartment, here in upper Manhattan to my offices in lower Manhattan.
Now, we all know that the shortest distance between two points is a straight line, but even if there's no traffic—a bit of a stretch even in our imaginary Manhattan—it'll still take us some amount of time to get there. By going faster and faster, we can reduce the travel time. But because nothing can go faster than the speed of light, there is a definite limit to how much time we can cut from our journey.
This Manhattan Universe fits with an old, classical vision of space, basically a flat grid that's static and unchanging. But when Albert Einstein looked at the fabric of space, he saw something completely different. He said that space wasn't static; it could warp and stretch.
And there could even be unusual structures of space called "wormholes." A wormhole is a bridge or tunnel that can link distant regions of space, in effect, a cosmic shortcut. In this kind of universe, my commute would be a New Yorker's dream.
But there's a hitch: to create a wormhole, you've got to rip or tear a hole in the fabric of space. But can the fabric of space really rip? Can this first step toward forming a wormhole actually happen? Well, you can't answer these questions on an empty stomach.
Turns out that by looking at my breakfast—coffee and a doughnut—we can get a pretty good sense of what string theory says about whether the fabric of space can tear.
Imagine that space is shaped like this doughnut. You might think that it would be very different from a region of space shaped like this coffee cup. But there's a precise sense in which the shape of the doughnut and the coffee cup are actually the same, just a little disguised. You see, they both have one hole. In the doughnut it's in the middle and in the coffee cup it's in the handle. That means we can change the doughnut into the shape of a coffee cup and back again without having to rip or tear the dough at all.
Okay, but suppose you want to change the shape of this doughnut into a very different shape, a shape with no holes. The only way to do that is to tear the doughnut like this and then re-shape it.
Unfortunately, according to Einstein's laws, this is impossible. They say that space can stretch and warp, but it cannot rip. Wormholes might exist somewhere fully formed, but you could not rip space to create a new one, over Manhattan or anywhere else. In other words, I can't take a wormhole to work.
But now string theory is giving us a whole new perspective on space, and it's showing us that Einstein wasn't always right. To see how, let's take a much closer look at the spatial fabric.
If we could shrink down to about a millionth of a billionth of our normal size, we'd enter the world of quantum mechanics, the laws that control how atoms behave. It's the world of light and electricity and everything else that operates at the smallest of scales. Here, the fabric of space is random and chaotic. Rips and tears might be commonplace. But if they were, what would stop a rip in the fabric of space from creating a cosmic catastrophe?
Well, this is where the power of strings comes in. Strings calm the chaos. And as a single string dances through space, it sweeps out a tube. The tube can act like a bubble that surrounds the tear, a protective shield with profound implications. Strings actually make it possible for space to rip.
Which means that space is far more dynamic and changeable than even Albert Einstein thought. So does that mean that wormholes are possible? Will I ever be able to take a stroll on Everest, grab a baguette in Paris and still make it back to New York in time for my morning meeting?
It would be kind of cool, though it's still a very distant possibility.
But one thing that is certain is that string theory is already showing us that the universe may be a lot stranger than any of us ever imagined. For example, string theory says we're surrounded by hidden dimensions, mysterious places beyond the familiar three-dimensional space we know.
AMANDA PEET: People who've said that there were extra dimensions of space, have been labeled as, you know, crackpots or people who are bananas. I mean, what, do you think there are extra dimensions? Well, string theory really predicts it.
BRIAN GREENE: What we think of as our universe could just be one small part of something much bigger.
SAVAS DIMOPOULOS: Perhaps we live on a membrane, a three-dimensional membrane that floats inside higher dimensional space.
BRIAN GREENE: There could be entire worlds right next to us, but completely invisible.
NIMA ARKANI-HAMED (Harvard University): These other worlds would, in a very literal sense, be, be parallel universes. This isn't a particularly exotic or, or strange notion.
BRIAN GREENE: No wonder physics students are lining up to explore the strange world of string theory.
SHELDON LEE GLASHOW: String theory is very active. Things are happening. There are a lot of people doing it. Most of the young kids, given the choice, at a ratio of something like ten to one, they will go into string theory.
BRIAN GREENE: But strings weren't always this popular. The pioneers of string theory struggled for years, working alone on an idea that nobody else believed in. Here's the gist of it: for decades, physicists believed that the tiniest bits inside an atom were point particles. Flying around the outside were the electrons, and inside were protons and neutrons which were made up of quarks. But string theory says that what we thought were indivisible particles are actually tiny, vibrating strings.
BURT OVRUT (University of Pennsylvania): It's nothing really mystical. It's a really tiny string. It either closes in to its little circle or it has end points, but it's just a little string.
BRIAN GREENE: In the 1980s, the idea caught on, and people started jumping on the string bandwagon.
MICHAEL B. GREEN: Well, the fact that suddenly all these other people were working in the field had its advantages and its disadvantages. It was wonderful to see how rapidly the subject could develop now, because so many people were working on it.
BRIAN GREENE: One of the great attractions of strings is their versatility. Just as the strings on a cello can vibrate at different frequencies, making all the individual musical notes, in the same way, the tiny strings of string theory vibrate and dance in different patterns, creating all the fundamental particles of nature. If this view is right, then put them all together and we get the grand and beautiful symphony that is our universe.
What's really exciting about this is that it offers an amazing possibility. If we could only master the rhythms of strings, then we'd stand a good chance of explaining all the matter and all the forces of nature, from the tiniest subatomic particles to the galaxies of outer space. This is the potential of string theory, to be a unified "Theory of Everything."
But, at first sight, in our enthusiasm for this idea, we seem to have gone too far. Because we didn't produce just one string theory, or even two—we somehow managed to come up with five.
MICHAEL DUFF (University of Michigan): Five different string theories, each competing for the title of the Theory of Everything.
BURT OVRUT: And if there's going to be a "The Fundamental Theory of Nature," there ought to be one of them.
AMANDA PEET: I suppose a number of string theorists thought, "Ah, that's fantastic. That's wonderful. And maybe one of these will end up being the right theory of the world." And yet, there must have been a little nagging voice at the back of the head that said, "Well, why are there five?"
BRIAN GREENE: With five competing players, the stage of string theory was looking a little crowded. The five theories had many things in common. For example, they all involved vibrating strings, but their mathematical details appeared to be quite different. Frankly, it was embarrassing. How could this unified Theory of Everything come in five different flavors?
This was a case where more was definitely less. But then something remarkable happened. This is Ed Witten. He's widely regarded as one of the world's greatest living physicists, perhaps even Einstein's successor.
MICHAEL B. GREEN: Ed Witten is a very special person in the field. He clearly has a grasp, particularly of the underlying mathematical principles, which is far greater than most other people.
JOSEPH POLCHINSKI (University of California, Santa Barbara): Well, you know, we all think we're very smart; he's so much smarter than the rest of us.
BRIAN GREENE: In 1995, string theorists from all over the world gathered at the University of Southern California for their annual conference. Ed Witten showed up at Strings 95 and rocked their world.
EDWARD WITTEN (Institute for Advanced Study): I was really trying to think of something that would be significant for the occasion. And actually, since five string theories was too many, I thought I would try to get rid of some of them.
BRIAN GREENE: To solve the problem, Witten constructed a spectacular new way of looking at string theory.
JOSEPH POLCHINSKI: Ed announced that he had thought about it, and moreover, he had solved it. He was going to tell us the solution to every string theory in every dimension, which was an enormous claim, but coming from Ed it was not so surprising.
BRIAN GREENE: The atmosphere was electric because, all of a sudden, string theory, which had been going through a kind of doldrums, was given an incredible boost, a shot in the arm.
LEONARD SUSSKIND (Stanford University): Ed Witten gave his famous lecture. And he said a couple of words that got me interested...and for the rest of the lecture...I got hooked up on the first few words that he said, and completely missed the point of his lecture.
NATHAN SEIBERG (Institute for Advanced Study): I remember I had to give the talk after him, and I was kind of embarrassed to.
JOSEPH LYKKEN: Ed Witten just blew everybody away.
BRIAN GREENE: Ed Witten blew everybody away because he provided a completely new perspective on string theory. From this point of view, we could see that there weren't really five different theories. Like reflections in a wall of mirrors, what we thought were five theories turned out to be just five different ways of looking at the same thing. String theory was unified at last.
Witten's work sparked a breakthrough so revolutionary that it was given it's own name, "M-theory," although no one really knows what the M stands for.
S. JAMES GATES, JR.: Aah, what is the M for?
BURT OVRUT: M-theory.
STEVEN WEINBERG (University of Texas at Austin): M-theory.
DAVID GROSS: M-theory.
JOSEPH LYKKEN: M-theory.
GARY HOROWITZ (Institute for Advanced Study): The M-theory.
STEVEN WEINBERG: M-theory is a theory...
BURT OVRUT: I don't actually know what the M stands for.
STEVEN WEINBERG: Well, the M has...
BURT OVRUT: I've heard many descriptions.
STEVEN WEINBERG: Mystery theory, magic theory...
JOSEPH LYKKEN: It's the Mother theory.
STEVEN WEINBERG: Matrix theory.
LEONARD SUSSKIND: Monstrous theory? I don't know what it...I don't know what Ed meant.
EDWARD WITTEN: M stands for magic, mystery or matrix, according to taste.
SHELDON LEE GLASHOW: I suspect that the "M" is an upside down "W" for "Witten."
EDWARD WITTEN: Some cynics have occasionally suggested that M may also stand for "murky," because our level of understanding of the theory is, in fact, so primitive. Maybe I shouldn't have told you that one.
BRIAN GREENE: Whatever the name, it was a bombshell. Suddenly everything was different.
JOSEPH LYKKEN: There was a lot of panic, if you like, realizing that big things were happening, and all of us not wanting to get left behind in this new revolution of string theory.
BRIAN GREENE: After Witten's talk, there was renewed hope that this one theory could be the theory to explain everything in the universe. But there was also a price to pay.
Before M-theory, strings seemed to operate in a world with 10 dimensions. These included one dimension of time, the three familiar space dimensions, as well as six extra dimensions, curled up so tiny that they're completely invisible.
GARY HOROWITZ: Well, we think these extra dimensions exist because they come out of the equations of string theory. Strings need to move in more than three dimensions. And that was a shock to everybody, but then we learned to live with it.
BRIAN GREENE: But M-theory would go even further, demanding yet another spatial dimension, bringing the grand total to 11 dimensions.
BURT OVRUT: We know that there would have to be 11 dimensions for this theory to make sense. So there must be 11 dimensions. We only see three plus one of them. How is that possible?
BRIAN GREENE: For most of us, it's virtually impossible to picture the extra, higher dimensions: I can't. And it's not surprising. Our brains evolved sensing just the three spatial dimensions of everyday experience. So how can we get a feel for them?
One way is to go to the movies.
THEATER BRIAN GREENE: We're all familiar with the real world having three spatial dimensions. That is, anywhere I go, I can move left-right, back-forth, or up-down.
MOVIE SCREEN BRIAN (on screen): But in the movies, things are a bit different. Even though the characters on a movie screen look three-dimensional, they actually are stuck in just two dimensions. There is no back-forth on a movie screen, that's just an optical illusion.
To really move in the back-forth dimension, I'd have to step out of the screen. And sometimes moving into a higher dimension can be a useful thing to do.
MOVIE SCREEN BRIAN GREENE (in theater): So dimensions all have to do with the independent directions in which you can move. They're sometimes called "degrees of freedom."
THEATER BRIAN GREENE: The more dimensions or degrees of freedom you have, the more you can do.
MOVIE SCREEN BRIAN GREENE (in theater): That's right.
BRIAN GREENE: And if there really are 11 dimensions, then strings can do a lot more, too.
BURT OVRUT: People found, fairly soon, that there were objects that lived in these theories, which weren't just strings, but were larger than that. They actually looked like membranes or surfaces.
BRIAN GREENE: The extra dimension Witten added allows a string to stretch into something like a membrane, or a "brane" for short. A brane could be three-dimensional or even more. And with enough energy, a brane could grow to an enormous size, perhaps even as large as a universe.
This was a revolution in string theory.
STEVEN WEINBERG: String theory has gotten much more baroque. I mean now there are not only strings, there are membranes. People go on calling this string theory, but the string theorists are not sure it really is a theory of strings anymore.
BRIAN GREENE: The existence of giant membranes and extra dimensions would open up a startling new possibility, that our whole universe is living on a membrane, inside a much larger, higher dimensional space.
It's almost as if we were living inside...a loaf of bread? Our universe might be like a slice of bread, just one slice, in a much larger loaf that physicists sometimes call the "bulk."
And if these ideas are right, the bulk may have other slices, other universes, that are right next to ours, in effect, "parallel" universes.
Not only would our universe be nothing special, but we could have a lot of neighbors. Some of them could resemble our universe, they might have matter and planets and, who knows, maybe even beings of a sort.
Others certainly would be a lot stranger. They might be ruled by completely different laws of physics. Now, all of these other universes would exist within the extra dimensions of M-theory, dimensions that are all around us. Some even say they might be right next to us, less than a millimeter away.
But if that's true, why can't I see them or touch them?
BURT OVRUT: If you have a brane living in a higher dimensional space, and your particles, your atoms, cannot get off the brane, it's like trying to reach out, but you can't touch anything. It might as well be on the other end of the universe.
JOSEPH LYKKEN: It's a very powerful idea because if it's right it means that our whole picture of the universe is clouded by the fact that we're trapped on just a tiny slice of the higher dimensional universe.
BRIAN GREENE: It is a powerful idea, especially because it may help solve one of the great mysteries of modern science. It has to do with gravity. It's been more than 300 years since Isaac Newton came up with the universal law of gravity, inspired, as the story goes, by seeing an apple fall from a tree. Today, it seems obvious that gravity is a powerful force.
SHELDON LEE GLASHOW: It would seem to most people that gravity is a very important force, it's very strong. It's very hard to get up in the morning, stand up, and when things fall they break because gravity is strong. But the fact of the matter is that it's not strong. It's, it's really a very weak force.
BRIAN GREENE: Gravity pulls us down to the Earth, and keeps our Earth in orbit around the sun. But in fact, we overcome the force of gravity all the time. It's not that hard. Even with the gravity of the entire Earth pulling this apple downward, the muscles in my arm can easily overcome it.
And it's not just our muscles that put gravity to shame. Magnets can do it, too, no sweat. Magnets carry a different force, the electromagnetic force. That's the same force behind light and electricity. It turns out that electromagnetism is much, much stronger than gravity.
Gravity, in comparison, is amazingly weak. How weak? The electromagnetic force is some thousand billion, billion, billion, billion times stronger. That's a one with 39 zeroes following it.
The weakness of gravity has confounded scientists for decades. But now, with the radical world of string theory, filled with membranes and extra dimensions, there's a whole new way to look at the problem.
NIMA ARKANI-HAMED: One way of approaching the question of why gravity is so weak compared to all the other forces, is to turn the question completely on its head, and say, "No, actually gravity isn't very weak. Compared to all the other forces, it just appears to be weak."
BRIAN GREENE: It may be that gravity is actually just as strong as electromagnetism, but for some reason, we can't feel its strength.
SAVAS DIMOPOULOS: Consider a pool table, a very large pool table. Think of the surface of the pool table as representing our three-dimensional universe, although it is just two-dimensional, and think of the billiard balls as representing atoms and other particles that the universe is made out of.
BRIAN GREENE: So here's the wild idea: the atoms and particles that make up stuff in the world around us will stay on our particular membrane, our slice of the universe just as the billiard balls will stay on the surface of the pool table—unless you're a really bad pool player.
But whenever the balls collide, there is something that always seeps off the table, sound waves. That's why I can hear the collision. Now, the idea is that gravity might be like the sound waves, it might not be confined to our membrane. It might be able to seep off our part of the universe.
Or think about it another way. Instead of pool tables, let's go back to bread. Imagine that our universe is like this slice of toast. And that you and me, and all of matter—light itself, everything we see—is like jelly. Now jelly can move freely on the surface of the toast, but otherwise, it's stuck, it can't leave the surface itself.
But what if gravity were different? What if gravity were more like cinnamon and sugar? Now, this stuff isn't sticky at all, so it easily slides right off the surface.
But why would gravity be so different from everything else that we know of in the universe? Well, turns out that string theory, or M-theory, provides an answer. It all has to do with shape.
For years, we concentrated on strings that were closed loops, like rubber bands. But after M-theory, we turned our attention to other kinds. Now we think that everything we see around us, like matter and light, is made of open-ended strings, and the ends of each string are tied down to our three-dimensional membrane.
But closed loops of string do exist, and one kind is responsible for gravity. It's called a graviton. With closed loops, there are no loose ends to tie down, so gravitons are free to escape into the other dimensions, diluting the strength of gravity and making it seem weaker than the other forces of nature.
This suggests an intriguing possibility. If we do live on a membrane and there are parallel universes on other membranes near us, we may never see them, but perhaps we could one day feel them through gravity.
SAVAS DIMOPOULOS: If there happens to be intelligent life on one of the membranes, then this intelligent life might be very close to us. So theoretically, and purely theoretically, we might be able to communicate with this intelligent life by exchanging strong gravity wave sources.
BRIAN GREENE: So who knows? Maybe someday we'll develop the technology and use gravity waves to actually communicate with other worlds.
ALIEN: Ay-yoo-ya.
BRIAN GREENE: Yes, hey, it's Brian. How you doing?
ALIEN: Brian, hoh-ba jubby wah-fa-loo poo-jabba "Simpsons!"
TRANSLATION: I told you never to call me during "The Simpsons!"
BRIAN GREENE: We don't really know if parallel universes could have a real impact on us. But there is one very controversial idea, which says they've already played a major role. In fact, it gives them credit for our existence.
As the classic story goes, the vast universe we see today was once extremely small, unimaginably small. Then, suddenly, it got bigger—a lot bigger—during the dramatic event known as the big bang.
The big bang stretched the fabric of space and set off the chain of events that brought us to the universe we know and love today. But there's always been a couple of problems with the big bang theory. First, when you squeeze the entire universe into an infinitesimally small, but stupendously dense package, at a certain point, our laws of physics simply break down. They just don't make sense anymore.
DAVID GROSS: The formulas we use start giving answers that are nonsensical. We find total disaster. Everything breaks down, and we're stuck.
BRIAN GREENE: And on top of this, there's the bang itself. What exactly is that?
ALAN GUTH: That's actually a problem. The classic form of the big bang theory really says nothing about what banged, what happened before it banged, or what caused it to bang.
BRIAN GREENE: Refinements to the big bang theory do suggest explanations for the Bang, but none of them turn the clock back completely to the moment when everything started.
PAUL STEINHARDT (Princeton University): Most people come at this with the naí¯ve notion that there was a beginning—that somehow space and time emerged from nothingness into somethingness.
BURT OVRUT: Well, I don't know about you, but I don't like nothing. Do I really believe that the universe was a big bang out of nothing? And I'm not a philosopher, so I won't say. But I could imagine to a philosopher, that is a problem. But to a physicist, I think, it's also a problem.
BRIAN GREENE: Everyone admits there are problems. The question is: "Can string theory solve them?" Some string theorists have suggested that the Big Bang wasn't the beginning at all, that the universe could have existed long before even forever. Not everyone is comfortable with the idea.
ALAN GUTH: I actually find it rather unattractive to think about a universe without a beginning. It seems to me that a universe without a beginning is also a universe without an explanation.
BRIAN GREENE: So what is the explanation? What if string theory is right, and we are all living on a giant membrane inside a higher dimensional space?
PAUL STEINHARDT: One of the ideas in string theory that was particularly striking to me, and suggested perhaps a new direction for cosmology, is the idea of branes and the idea of branes moving in extra dimensions.
BRIAN GREENE: Some scientists have proposed that the answer to the Big Bang riddle lies in the movements of these giant branes.
BURT OVRUT: It's so simple. Here's a brane on which we live, and here's another brane floating in the higher dimension. There's absolutely nothing difficult about imagining that these collide with each other.
BRIAN GREENE: According to this idea, some time before the big bang, two branes carrying parallel universes began drifting toward each other, until...
BURT OVRUT: All of that energy has to go somewhere. Where does it go? It goes into the big bang. It creates the expansion that we see, and it heats up all the particles in the universe in this big, fiery mass.
BRIAN GREENE: As if this weren't weird enough, the proponents of this idea make another radical claim: the big bang was not a special event.
They say that parallel universes could have collided, not just once in the past, but again and again—and that it will happen in the future. If this view is right, there's a brane out there right now, headed on a collision course with our universe.
PAUL STEINHARDT: So another collision is coming, and there'll be another big bang. And this will just repeat itself for an indefinite period into the future.
BRIAN GREENE: It's an intriguing idea. Unfortunately, there are a few technical problems.
DAVID GROSS: Well, that was a very ingenious scenario that arose naturally within string theory. However, the good old problems creep back in again.
BRIAN GREENE: The fact is we don't really know what happens when two branes collide. You can wind up with the same situation we had with the Big Bang; the equations don't make sense.
GARY HOROWITZ: They have to make a lot of assumptions in their models, and I don't think they've really solved the problem of the big bang in string theory.
BRIAN GREENE: If string theory is the one true theory of the universe, it will have to solve the riddle of the big bang. And there's a lot of hope that someday string theory will succeed. But for now, there's also a lot of uncertainty. As promising and exciting the theory is, we don't entirely understand it.
DAVID GROSS: It's as if we've stumbled in the dark into a house, which we thought was a two bedroom apartment and now we're discovering is a nineteen-room mansion—at least. And maybe it's got a thousand rooms, and we're just beginning our journey.
BRIAN GREENE: So how sure are we that the universe is the way that string theory describes it? Is the world really made up of strings and membranes, parallel universes and extra dimensions? Is this all science or science fiction?
MICHAEL DUFF: Well, the question we often ask ourselves as we work through our equations is, "Is this just fancy mathematics, or is it describing the real world?'
S. JAMES GATES, JR.: These exercises in our imagination of mathematics are all, at the end of the day, subjected to a single question: "Is it there in the laboratory? Can you find its evidence?"
JOSEPH LYKKEN: String theory and string theorists do have a real problem. How do you actually test string theory? If you can't test it in the way that we test normal theories, it's not science, it's philosophy, and that's a real problem.
BRIAN GREENE: Strings are thought to be so tiny, much smaller than an atom, that there's probably no way to see them directly. But even if we never see strings, we may someday see their fingerprints. You see, if strings were around at the beginning of the universe, when things were really tiny, they would have left impressions or traces on their surroundings. And then, after the big bang, when everything expanded, those traces would have been stretched out along with everything else. So, if that's true, we may someday see the tell-tale signs of strings somewhere in the stars.
But even here on earth there's a chance we can detect evidence of strings. This pasture in Illinois serves as command central for a lot of this research. Well, actually, the real work happens underground where the hunt is on for evidence supporting string theory, including extra dimensions.
JOSEPH LYKKEN: Not too many years ago, people who talked about large extra dimensions would have been considered crackpots, to put it lightly.
BRIAN GREENE: But all that has changed, thanks to string theory.
This is Fermilab, and right now, it's our best hope for proving that extra dimensions are real. Fermilab has a giant atom smasher. Here's how it works: scientists zap hydrogen atoms with huge amounts of electricity. Later, they strip them of their electrons and send the protons zooming around a four mile circular tunnel beneath the prairie. Just as they're approaching the speed of light, they are steered into collisions with particles whizzing in the opposite direction.
Most collisions are just glancing blows, but occasionally there's a direct hit. The result is a shower of unusual subatomic particles. The hope is that among these particles will be a tiny unit of gravity, the graviton.
Gravitons, according to string theory, are closed loops, so they can float off into the extra dimensions. The grand prize would be a snapshot of a graviton at the moment of escape.
MARIA SPIROPULU (Fermilab): And then the graviton goes to the extra dimension, and then it shows in the detector by its absence. You see it by its absence.
BRIAN GREENE: Unfortunately, Fermilab hasn't yet "seen" the vanishing graviton. And the pressure is on, because another team is hot on the same trail. Four thousand miles away, on the border of France and Switzerland, a lab called CERN is constructing an enormous new atom smasher. When it's finished, it will be seven times more powerful than Fermilab's.
JOSEPH LYKKEN: There's a great sense of urgency that every minute has to count, but eventually, CERN, our rival laboratory, will frankly blow us out of the water.
BRIAN GREENE: CERN will blow Fermilab out of the water, not only in the search for extra dimensions, but other wild ideas.
At the top of the "to do" list for both labs is the hunt for something called "supersymmetry," that's a central prediction of string theory. And it says, in a nutshell, that for every subatomic particle we're familiar with, like electrons, photons, and gravitons, there should also be a much heavier partner called a "sparticle," which so far no one has ever seen.
Now, because string theory says sparticles should exist, finding them is a major priority.
MARIA SPIROPULU: So, it's a big discovery to find supersymmetry. That's, that's a humongous discovery and, and I think it's a bigger discovery to find supersymmetry than to find life on Mars.
AMANDA PEET: If we were to hear tomorrow that supersymmetry was discovered, there would be parties all over the planet.
BRIAN GREENE: The problem is, if they exist, the sparticles of supersymmetry are probably incredibly heavy, so heavy that they may not be detected with today's atom smashers. The new facility at CERN will have the best chance, once it's up and running in several years, but that won't stop the folks at Fermilab from trying to find them first.
MARIA SPIROPULU: The competition is, is friendly and fierce at the same time. We're competing like bad dogs, essentially. It has always been like that, and it will always be like that.
JOSEPH LYKKEN: We have to make sure that we don't make any mistakes, that we do absolutely the best we can do at these experiments and take advantage of what is really one of the great golden opportunities for discovery.
BRIAN GREENE: If we do find sparticles, it won't prove string theory, but it will be really strong circumstantial evidence that we're on the right track. Over the next 10 to 20 years, the new generation of atom smashers is sure to uncover surprising truths about the nature of our universe.
But will it be the universe predicted by string theory? What if we don't find sparticles? Or extra dimensions? What if we never find any evidence that supports this weird new universe filled with membranes and tiny vibrating strings? Could string theory, in the end, be wrong?
MICHAEL DUFF: Oh yes, it's certainly a logical possibility that we've all been wasting our time for the last twenty years and that the theory is completely wrong.
JOSEPH LYKKEN: There have been periods of many years where all of the smart people, all of the cool people, were working on one kind of theory, moving in one kind of direction, and even though they thought it was wonderful, it turned out to be a dead end. This could happen to string theory.
BRIAN GREENE: Even though there's no real evidence yet, so much of string theory just makes so much sense; a lot of us believe it's just got to be right.
STEVEN WEINBERG: I don't think it's ever happened that a theory that has the kind of mathematical appeal that string theory has turned out to be entirely wrong. I would find it hard to believe that that much elegance and mathematical beauty would simply be wasted.
GARY HOROWITZ: I don't really know how close we are to the end. You know, are we almost there in having the complete story? Is it going to still be another ten years? Nobody knows. But I think it's going to keep me busy for a long time.
JOSEPH LYKKEN: We have been incredibly lucky. Nature has somehow allowed us to unlock the keys to many fundamental mysteries already. How far can we push that? We won't know until we, until we try.
BRIAN GREENE: A century ago, some scientists thought they had pretty much figured out the basic laws of the universe. But then Einstein came along and dramatically revised our views of space and time and gravity. And quantum mechanics unveiled the inner workings of atoms and molecules, revealing a world that's bizarre and uncertain.
So, far from confirming that we had sorted it all out, the 20th century showed that every time we looked more closely at the universe, we discovered yet another unexpected layer of reality.
As we embark on the 21st century, we're getting a glimpse of what may be the next layer: vibrating strings, sparticles, parallel universes and extra dimensions. It's a breathtaking vision, and in a few years, experiments may begin to tell us whether some of these ideas are right or wrong.
But, regardless of the outcome, we'll keep going, because, well, that's what we do. We follow our curiosity. We explore the unknown. And a hundred or a thousand years from now, today's view of the cosmos may look woefully incomplete, perhaps even quaint. But undeniably, the ideas we call string theory are a testament to the power of human creativity. They've opened a whole new spectrum of possible answers to age-old questions. And with them, we've taken a dramatic leap in our quest to fully understand this elegant universe.
On NOVA's Website, go behind the scenes with Brian Greene. Journey into the subatomic world, play with strings, picture other dimensions and much more. Find it on PBS.org.
To order this program on VHS or DVD, or the book, The Elegant Universe, please call WGBH Boston Video at 1-800-255-9424.
Next time on NOVA, a century after the Wright Brothers took flight, modern builders attempt to recreate the world's first airplane, but will their dreams take off? Wright Brothers' Flying Machine.
NOVA is a production of WGBH Boston.
Broadcast Credits
The Elegant Universe: Part 3
- Hosted by
- Brian Greene
- Based on
- The Elegant Universe by Brian Greene
-
Edited by
(Einstein's Dream and String's the Thing) - Jonathan Sahula
-
Edited by
(Welcome to the 11th Dimension) - Dick Bartlett
-
Written, Produced and Directed by
(Einstein's Dream and String's the Thing) - Joseph McMaster
-
Written, Produced and Directed by
(Welcome to the 11th Dimension) -
Julia Cort
Joseph McMaster - Series Producer and Director
- David Hickman
- Series Coordinating Producer
- Andrea Cross
- Series Producer
- Joseph McMaster
- Director of Photography
- Mike Coles
- Sound Recordist
- Keith Rodgerson
- Assistant Camera
- Richard Comrie
- Animation
-
Edgeworx, Inc.
Red Vision -
Visual Effects Supervisors
(Einstein's Dream and String's the Thing) -
John Bair
Jonathan Sahula -
Visual Effects Supervisors
(Welcome to the 11th Dimension) -
John Bair
Dick Bartlett - Music composed by
- Ed Tomney
- Additional Music
- Asche & Spencer
-
Associate Producers
(Einstein's Dream and String's the Thing) -
Barbara Park
Marty Johnson
Jennifer Callahan
Rob Meyer -
Associate Producers
(Welcome to the 11th Dimension) -
Barbara Park
Marty Johnson
Rob Meyer
Julie Crawford
Jennifer Callahan
-
Additional Editing
(Einstein's Dream) -
Dick Bartlett
Rebecca Nieto -
Additional Editing
(String's the Thing) - Rebecca Nieto
-
Additional Editing
(Welcome to the 11th Dimension) -
Jonathan Sahula
Rebecca Nieto -
Additional Camera
(Einstein's Dream and String's the Thing) - Brian Dowley
-
Additional Camera
(Welcome to the 11th Dimension) - James Callanan
-
Studio Art Director
(Einstein's Dream and String's the Thing) - Sabina Sattar
-
Set Builders
(Einstein's Dream and String's the Thing) -
Alistair Bell
Jason Chalmers -
Extras Costume Designer
(Einstein's Dream and String's the Thing) - Agnes Treplin
-
Make-up
(Einstein's Dream and String's the Thing) -
Sarah Berry
Tony Lilley -
Cellist
(Einstein's Dream and String's the Thing) - Kate Sawbridge
-
Cellist
(Welcome to the 11th Dimension) - Nicole Cariglia
-
Production Managers, London
(Einstein's Dream and String's the Thing) -
Jane Moss
Tessa Pemberton -
Production Managers, London
(Welcome to the 11th Dimension) -
Jane Moss
Tessa Pemberton
Catherine Alen-Buckley - Online Editor
- David Tecson
- Colorist
- Greg Dildine, Finish Editorial
-
Audio Mix
(Einstein's Dream and String's the Thing) -
Heart Punch Studio
John Jenkins, WGBH -
Audio Mix
(Welcome to the 11th Dimension) - John Jenkins, WGBH
-
Archival Material
(Einstein's Dream) -
AIP Emilio Segrè Visual Archives
AP / Wide World Photos
BPK, Berlin
California Institute of Technology
Philippe Halsman, Halsman Estate
Image Archive of the ETH-Library, Zurich
Image Bank Film / Archive Films / Getty Images
Library of Congress, Prints & Photographs Division
Lotte Jacobi Collection, University of New Hampshire
Lucien Aigner ©The Lucien Aigner Trust
Manoogian Collection
NASA and Space Telescope Science Institute
National Archives and Records Administration
Pittsburgh Post-Gazette
Science Museum / Science & Society Picture Library
ullstein bild Berlin
Underwood & Underwood / CORBIS -
Archival Material
(String's the Thing) -
BPK, Berlin
Brown Brothers, Sterling, PA
CERN
The New York Times
SCIENCE
Sveriges Television AB -
Archival Material
(Welcome to the 11th Dimension) -
AP / Wide World Photos
Image Bank Film/Archive Films/Getty Images
Science Museum/Science & Society Picture Library
ullstein bild Berlin -
Special Thanks
(Einstein's Dream) -
Promotional Sponsor SEED Magazine
John Charap
The Forrest Family
Andrew J. Hanson
Institute for Advanced Study
Kodak Entertainment Imaging
Light Street, LLC
The Maskin Family
Muse Hotel, New York
Gretchen Sinnett
Georgia Whidden -
Special Thanks
(String's the Thing) -
Promotional Sponsor SEED Magazine
Blue Night Records
The Forrest Family
Andrew J. Hanson
Institute for Advanced Study
Kodak Entertainment Imaging
Light Street, LLC
Muse Hotel, New York
Gretchen Sinnett
Georgia Whidden -
Special Thanks
(Welcome to the 11th Dimension) -
Promotional Sponsor SEED Magazine
The Forrest Family
Institute for Advanced Study
Kodak Entertainment Imaging
Light Street, LLC
Muse Hotel, New York
Georgia Whidden
White Sands Space Harbor
- Advisors
-
Melissa Franklin, Harvard University
Peter Galison, Harvard University
Jim Gates, University of Maryland
Paul Hickman, CESAME, Northeastern University
Joseph Polchinski, University of California, Santa Barbara
Frederick Stein, American Physical Society
Paul Steinhardt, Princeton University
Edwin Taylor, Massachusetts Institute of Technology
Stamatis Vokos, Seattle Pacific University
Steven Weinberg, University of Texas at Austin - NOVA Series Graphics
- National Ministry of Design
- NOVA Theme
-
Mason Daring
Martin Brody
Michael Whalen -
Post Production Online Editors
(Einstein's Dream and String's the Thing) -
Spencer Gentry
Mark Steele -
Post Production Online Editor
(Welcome to the 11th Dimension) - Spencer Gentry
- Closed Captioning
- The Caption Center
- NOVA Administrator
- Queene Coyne
- Publicity
-
Jonathan Renes
Diane Buxton
Tom Stebbins - Senior Researcher
- Ethan Herberman
- Production Coordinator
- Linda Callahan
- Unit Managers
-
Holly Archibald
Lola Norman-Salako - Paralegal
- Nancy Marshall
- Legal Counsel
- Susan Rosen Shishko
- Post Production Assistant
- Patrick Carey
- Associate Producer, Post Production
- Nathan Gunner
- Post Production Supervisor
- Regina O'Toole
- Post Production Editor
- Rebecca Nieto
- Post Production Manager
- Maureen Barden Lynch
- Supervising Producers
-
Lisa D'Angelo
Stephen Sweigart - Producer, Special Projects
- Susanne Simpson
- Coordinating Producer
- Laurie Cahalane
- Senior Science Editor
- Evan Hadingham
- Senior Series Producer
- Melanie Wallace
- Managing Director
- Alan Ritsko
- Senior Executive Producer
- Paula S. Apsell
A NOVA Production for WGBH/Boston and Channel 4 in association with David Hickman Films, Sveriges Television and Norddeutscher Rundfunk
© 2003 WGBH Educational Foundation
All rights reserved
Any opinions, findings, and conclusions or recommendations expressed in this material are those of the author(s) and do not necessarily reflect the views of the National Science Foundation.
- Image credit: (image composite) © WGBH/NOVA
Preview
Notice: Undefined index: preview_video_length in /home/nova/zend/application/templates/resources/multi-ep-pro.phtml on line 497
Full Program | 53:51
Full program available for streaming through ...
Watch Online
Full program available
Soon