 |
 |
 |
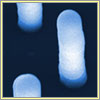
| |
Introduction
Think small—really small. Nanotechnology employs devices with
dimensions of one to 1,000 nanometers. To put this in perspective, consider the
following: a nanometer is 1/80,000th the width of a human hair; it is the
length of 10 hydrogen atoms placed end to end; and it is less than one third
the height of a single twist on a strand of DNA. At these sizes it is no wonder
that scientists have seized upon nanotechnology for myriad medical
applications. After all, the human body is built upon a foundation of
nature-made nanostructures—genes, proteins, cells—that may be best
approached on their own scale. Cancer is the key area of medical nanotechnology
research. In the not too distant future, dozens of intriguing nanodevices such
as the nanotubes at left may transform cancer diagnosis, treatment, and
prevention.—Lexi Krock
|
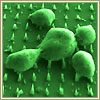
| |
Nanotubes
Nanotubes are hollow cylinders made of carbon atoms. Doctors could someday use
them as miniscule syringes for injecting cells with drugs from within the body,
or as nanoscale diagnostic probes the patient would never feel. They can also
be filled and sealed, forming test tubes or potential drug delivery devices.
Here, an array of nanotubes engage in what their creators at the Oak Ridge
National Laboratory call "impalefection," the capturing of genetic materials by
impaling cells (a hamster's ovary cells in this case). In the future, nanotubes
could help identify DNA mutations associated with a risk of cancer.
|
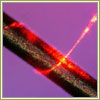
| |
Nanowire
This glowing silica nanowire is wrapped around a single strand of human hair.
It looks delicate—it is about five times smaller than a virus—but
it is several times stronger than spider silk. Researchers have developed
coated nanowires that bind to certain proteins that can indicate the presence
of prostate cancer before conventional tests can. Other potential applications
for nanowires include the early sensing of breast and ovarian malignancies.
Nanowires are so small that doctors could one day implant them into the body as
permanent health detectives that continuously monitor molecular levels.
|
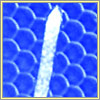
| |
Nanocantilever
The honeycomb mesh behind this tiny carbon cantilever is the surface of a fly's
eye. Cantilevers are beams anchored at only one end. In the nanoworld, they
function as sensors ideal for detecting the presence of extremely small
molecules in biological fluids. Arrays of nanocantilevers coated with
antibodies, for example, will bend from the changes in surface tension when
substrates that signal a malignancy bind to it. Simply by monitoring whether or
not such nanocantilevers are bent, specialists may someday be able to identify
the presence of cancer molecules that today are difficult to detect.
|
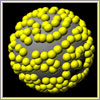
| |
Nanoshells
Nanoshells are hollow silica spheres covered with gold. Scientists can attach
antibodies to their surfaces, enabling the shells to target certain cells such
as cancer cells. In mouse tests, Naomi Halas's research team at Rice University
directed infrared radiation through tissue and onto the shells, causing the gold to
superheat and destroy tumor cells while leaving healthy ones intact.
Technicians can control the amount of heat with the thickness of the gold
and the kind of laser. Nanoshells
could one day also be filled with drug-containing polymers. Heating them would
cause the polymers to release a controlled amount of the drug. Human trials
using gold nanoshells are slated to begin in a couple of years.
|
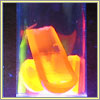
| |
Quantum Dots
Quantum dots are miniscule semiconductor particles that can serve as signposts
of certain types of cells or molecules in the body. They can do this because
they emit different wavelengths of radiation depending on the type of cadmium
used in their cores: cadmium sulfide for ultraviolet to blue, cadmium selenide
(seen here) for most of the visible spectrum, and cadmium telluride for the far
red and near-infrared. (A dot's size determines its precise color within each
range.) A polymer coating enables researchers to attach molecules such as
antibodies that will seek out and attach to tumors and other targeted cells.
The coating also shields nearby cells from the cadmium's toxicity. The
different colors of quantum dots provide a powerful tool for labeling and
monitoring multiple cells and molecules simultaneously.
|
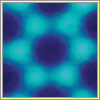
| |
Nanopores
Nanopores have cancer research and treatment applications. Engineered into
particles, they are holes that are so tiny that DNA molecules can pass through
them one strand at a time, allowing for highly precise and efficient DNA
sequencing. As a DNA strand moves through a nanopore, scientists can monitor
each "letter" on it, deciphering coded information, including mutations
associated with cancer. By engineering nanopores into the surface of a drug
capsule that are only slightly larger than the medicine's molecular structure,
drug manufacturers can also use nanopores to control the rate of a drug's
diffusion in the body.
|
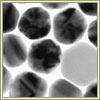
| |
Gold Nanoparticles
These nanoparticles, seen in a transmission electron micrograph image, are
similar in structure to nanoshells, but they have a solid core. Researchers at
Northwestern University are using gold nanoparticles to develop ultrasensitive
detection systems for DNA and protein markers associated with many forms of
cancer, including breast and prostate cancer. The scientists can release swarms
of nanoparticles linked to a host of cancer-related antibodies. The
nanoparticles can hunt for hundreds of different cancer targets simultaneously.
Their tests with cancer molecules in solution revealed that gold nanoparticles
are up to one million times as sensitive as conventional cancer-detection
approaches.
|
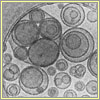
| |
Liposomes
Liposomes—tiny pouches made of lipids, or fat molecules, surrounding a
water core—were the first type of nanoparticles widely used for clinical
cancer treatment. Several different kinds of liposomes are also widely employed
against infectious diseases and can deliver certain vaccines. During cancer
treatment they encapsulate drugs, shielding healthy cells from their toxicity,
and prevent their concentration in vulnerable tissues such as those of a
patient's kidneys and liver. Liposomes can also reduce or eliminate certain
common side effects of cancer treatment such as nausea and hair loss.
|
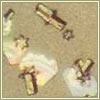
| |
Fullerenes
These crystalline particles are a form of carbon atom whose molecular
architecture is arranged in a soccer ball-like structure. Also known as
buckyballs, they were discovered in 1985 among the detritus of laser-vaporized
graphite. Unlike other molecules that have applications as cancer drug delivery
vehicles, fullerenes don't break down in the body and are excreted intact. This
trait can be important for some cancer treatment compounds that are dangerous
to healthy cells. For example, fullerene drug delivery particles that contain
radioactive atoms would allow for the complete removal of radiation from the
body following treatment.
|
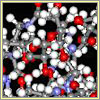
| |
Dendrimer
This fascinating particle holds significant promise for cancer treatment. Its
many branches allow other molecules to easily attach to its surface.
Researchers at the University of Michigan have fashioned dendrimers into
sophisticated anti-cancer machines carrying five chemical tools—a
molecule designed to bind to cancer cells, a second that fluoresces upon
locating genetic mutations, a third to assist in imaging tumor shape using
X-rays, a fourth carrying drugs released on demand, and a fifth that would send
a signal when cancerous cells are finally dead. The creators of these
dendrimers have had successful tests with cancer cells in culture and plan to
try them in living animals soon.
|