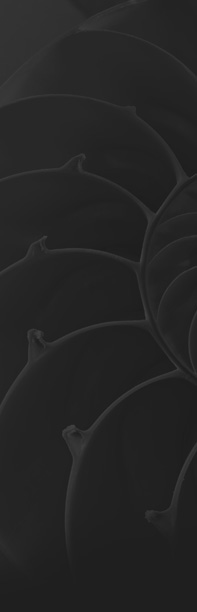
Making Stuff: Stronger
David Pogue tests his mettle against the world's strongest stuff, from steel and Kevlar to bioengineered silk. Airing August 21, 2013 at 9 pm on PBS Aired August 21, 2013 on PBS
- Originally aired 01.19.11
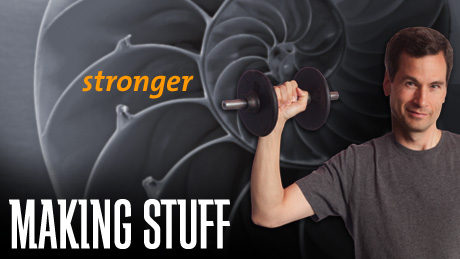
Program Description
Transcript
Making Stuff: Stronger
PBS Airdate: January 19, 2011
DAVID POGUE (Technology Reporter, New York Times) Imagine a world with smart buildings that can ride out any disaster; bacteria that make gasoline out of thin air; computers that think and place all the world's knowledge in your pocket; an end to surgery, with tiny devices that can repair cells, even D.N.A.; gossamer threads, strong enough hold up a bridge; or an elevator to the stars. These visions of the future are all based in the discoveries of today, as a new science of materials emerges from the elemental building blocks of the universe, promising a future in which we can create virtually anything we want, atom by atom.
Can I have some rock music please?
I'm David Pogue, and I am on a quest to find the world's most advanced materials.
A narrow wire that can bring a fighter jet to a dead stop in less than three seconds...
Welcome to Navy Airlines!
...super strong, but not unbreakable. What new material might replace it?
A manmade fiber that can stop a bullet?
Whoa!
Nature is our guide in this quest.
These are your lab assistants?
Inspired by the beak of a tropical bird.
Er, they were all out of parrots.
Or a lightweight thread spun by spiders, now made from goats' milk.
So this one's skim and this is two percent?
It's stronger than steel!
We're learning how to make stuff stronger than ever, and it's going to change the world.
Marc Meyers (Materials Scientist, University of California, San Diego): You should try, too.
DAVID POGUE: Right now,...
Well it would snap if I had any upper body strength.
...on NOVA.
Here I am, inside a Navy plane. I'm on a quest to find the strongest stuff in the world. We're headed for that: the U.S.S. John C. Stennis. I can't believe it's possible to land on that thing.
But down on that tiny deck, there's a lifesaving piece of equipment that's supposed to be strong enough to bring our plane to a dead stop. Man, I hope that thing holds!
We're going down.
Wow, I really felt that! Now I want to see how it works.
Welcome to Navy Airlines!
Luckily, I've arrived at the perfect time, because a dozen new pilots are practicing their landings. That means lots of attempts to catch that wire.
There are actually four cables across the deck. They're working on number three right now. So this guy's going to try to catch number four. That's the ideal one. Let's see if he does it.
The jets land under full power, briefly exerting hundreds of thousands of pounds of force on a cable that's only an inch and a half thick. How is it possible that such a thin wire can have so much strength? I head below deck to get a closer look.
So, I think I understand how these planes land, because I came in on one of these planes. The plane has a hook underneath, and then there's this big steel rubber band on the deck that stretches a little bit, then un-stretches. Am I right?
Scot Vannorman (United States Navy): No, sir. It looks like that, yes, it does, but that actual rubber band that you're talking about, the actual wire, comes down here and actually leaves from here. This is actually the cable that you're going to see going across that flight deck.
DAVID POGUE: Every time a plane hits the cable, this powerful hydraulic piston goes into action. By spooling out wire at just the right speed it counteracts the momentum to slow the aircraft. The bigger the plane, the harder it pulls. It's designed to withstand a truly massive load.
SCOT VANNORMAN: The breaking strength of the cable is 215,000 pounds.
DAVID POGUE: That's enough strength to stop four F18s at once. Amazing! And it's all thanks to a strong material that the Navy trusts above all others.
The entire ship is made of steel.
Don't believe me; tell it to the magnet.
Steel.
Steel provides the structural strength of the bulkheads, and the decks.
Steel!
And, of course, the all-important arresting cable.
In the quest to find strong materials, steel is a great place to start. Our modern civilization is built on the stuff, but where does it come from?
Thousands of years ago, ancient people used tools made of stone. That's why it's called the "Stone Age." But, eventually, they discovered how to extract metals like copper, tin and iron from rocks. Iron is the main ingredient in steel, but steel is stronger than iron, because it also contains carbon.
All these materials fill the periodic table of the elements, a master chart that that lists the basic building blocks of every material in the world, from the lightest to the heaviest, from the weakest to the strongest.
The ability withstand pulling forces is called tensile strength. And steel has it in abundance. But it's not unbreakable.
Each time a plane hits the wire, the impact takes a toll, with potentially disastrous results. In 2003, this fighter had nearly come to a complete stop when the cable suddenly snapped. The plane was lost but the pilot ejected to safety. Sudden failures like these are rare, because the crew doesn't wait for the cables to wear out.
So the doubled up cable down here on the pulleys, you, you replace that how often?
SCOT VANNORMAN: Every two thousand arrestments.
DAVID POGUE: And, they're connected to one across the deck up there that has to be replaced every...
SCOT VANNORMAN: One hundred and twenty five.
DAVID POGUE: How come so much more often?
SCOT VANNORMAN: Well, the one on the deck—we call it the cross-deck pennant or C.D.P.—the C.D.P. takes the blunt force of the tailhook: metal-to-metal contact at a hundred and thirty to a hundred and forty miles per hour, a hundred and twenty-five times.
DAVID POGUE: It's amazing that they haven't found some material in the whole world that would last more than a hundred and twenty five landings.
SCOT VANNORMAN: I don't know of any metal that can do that yet.
DAVID POGUE: Steel has incredible tensile strength, but can it be improved?
Can we make this stuff even stronger?
That's what Ellwood Steel has tried to do for the last century. They've developed and produced high-strength steel for everything from aircraft crankshafts in World War II to high-performance windmill generator shafts and other steel components. It's all based on the same basic materials, first combined thousands of years ago.
John Paules (General Manager, Ellwood Technologies): The end product, steel, is a mostly iron—99 percent or so—iron with a little bit of carbon. First we melt it, and then we refine it. We remove all the impurities we can to get the properties that we need from the steel.
DAVID POGUE: At this plant, the raw material is scrap steel, to be recycled. First it's loaded into an electric furnace for melting.
When the mix reaches 3,000 degrees Fahrenheit, the liquid metal pours out of the bottom of the furnace into a ladle. Today, they're making steel for jumbo jet landing gear, extremely strong and stiff. Besides iron and carbon, the recipe calls for several additional elements, added in precise quantities. After cooling, a simple test reveals whether the steel has the right amount of tensile strength.
John Paules: When we pull a piece of steel, we measure how much it stresses. We keep increasing the load; we watch it stretch, until it eventually breaks.
DAVID POGUE: The more force it can withstand, the greater the tensile strength.
Where does steel's remarkable strength come from? In metals like iron, atoms are packed together like sheets of marbles. But when pushed or pulled, they can slip and slide past each other.
But steel also contains carbon. The carbon atoms fit in between the iron atoms, limiting their freedom of movement, making it harder to pull them apart. Steel makers long ago learned that by adding more carbon, you can increase the steel's tensile strength, but you give up flexibility, which is something that the arresting cable needs to wind and unwind. The trick is to find just the right balance.
John Paules: Including steel, there's always been a tradeoff between very high hardness, high strength and flexibility. Steel has provided a very good combination of high strength and good flexibility for many applications. That's why it's so widely used.
DAVID POGUE: Without steel's tensile strength to resist fracture, elevator cables would snap and bridges would crack in two. That alone makes it an incredibly valuable strong material. But steel protects us in other ways too. Carmakers depend on a different kind of strength in steel, which is on display at the Outlaw Motor Speedway in Muskogee, Oklahoma.
Materials scientist Mark Eberhart is here to help me understand what makes car bodies strong. And to do that that, we first have to watch them break.
Mark, you're a prominent scientist, author, teacher. Why are we at a dirt track in Oklahoma?
Mark Eberhart (Metallurgist, Author, Why Things Break): I've been fascinated with understanding why things break since I was about six years old. You can go into a lab, and you can do real detailed experiments—they don't capture the full beauty of why things break. This is the place to come to really understand what a person means when they say strength.
DAVID POGUE: We're the Starsky and Hutch of science nerds.
Can I have some rock music please?
Frankly, I wouldn't mind watching from the stands, but Mark insists that only by becoming crash test dummies ourselves can we understand how steel makes car bodies strong.
Mark Eberhart: Most people don't think of strength as kind of a monolithic thing, but it really is a combination...
DAVID POGUE: Hey, what's your hurry pal? Sorry about that.
Mark Eberhart: It's really a combination of properties. It's not just one property, the way most people use the word.
DAVID POGUE: So, really, when we say "strong as steel," it's a little more complicated than that?
Mark Eberhart: Oh, absolutely.
DAVID POGUE: Besides tensile strength, resistance to pulling, there's also toughness. It's a measure of how much energy a material can absorb without breaking.
When a car crashes and the body dents, the steel is absorbing the energy of the crash. That's toughness.
During our 100 laps the car took some serious hits. Did the steel demonstrate toughness?
You know it's not all that much different from Manhattan at rush hour. Let's check this puppy out.
Mark Eberhart: There's some damage over here.
DAVID POGUE: The body got creamed, but, the steel didn't break. It's dented, yet still intact. Our driver, Danny, suggests an even more extreme test of steel's lifesaving toughness.
So what do you got set up for us here?
Danny Womack (Owner, Outlaw Motor Speedway): We're going to take this car, we're going to send it into the wall and see what we can tear up.
DAVID POGUE: Danny locks the steering wheel in place and opens the throttle. Now it's up to me to do the honors.
In the name of science!
We installed a small camera where the driver would normally be.
Danny Womack: Ha ha. That was great.
DAVID POGUE: The impact was far more intense than anything we experienced during the race.
Mark, talk us through what's going on here.
Mark Eberhart: What we can see here is that we've exhausted a lot of the energy and just crumpling this thing up. I think we knocked the axle all the way back. Tell me, is that the case, Danny?
Danny Womack: It looks like it.
Mark Eberhart: It knocked it all the way back into the firewall.
Danny Womack: That deal'll buff right out.
DAVID POGUE: Would you have lived, Danny?
Danny Womack: I would have had a heart attack somewhere between there and here. I don't know.
DAVID POGUE: Yeah.
Amazingly the car took everything we threw at it. If the material hadn't been tough, if it had been too brittle, the damage would have been lethal. But in this case, people would have survived, because the steel absorbed the energy of the collision. The amount of carbon in the steel is low enough to let the atoms move just a bit, allowing the steel to bend but remain intact. That's toughness.
You know, who says science can't be fun? That was pretty cool.
Steel makes the biggest structures on Earth possible. And in the future, when buildings reach a mile or more into the sky and bridges stretch across the sea, steel will be there to support humanity's dreams, because we haven't come close to exhausting the potential of this most precious metal. But no material is perfect. Even metals have their weaknesses.
Consider the suit of armor. Warriors once wore bronze, iron and steel onto the battlefield. Sure it was heavy, but it provided good protection, until around the 15th century. When the gun appeared, heavy armor became a liability.
So, for hundreds of years, infantry in the world's bloodiest wars went to battle with little to protect them, because the metal was no match for a bullet. Why?
Luckily, I have a personal connection to a place where we can see exactly how metals fail under fire.
This has got to be my favorite spot at M.I.T., Strobe Alley, dedicated to the work of my great-uncle Harold Edgerton, a pioneer in the art of high-speed photography.
I remember, when I was a kid, he was always showing us pictures of what he was doing. He was smashing light bulbs, shooting bullets through apples, stuff like that. I thought, "That's the coolest job in the world."
Our plan today is to fire small, .22 caliber bullets at several different types of metal. Using a high-speed video camera, we'll examine the exact point when the bullet hits and we'll examine the type of hole it creates.
Jim Bales, who runs Strobe Alley will be our triggerman.
Jim Bales (Edgerton Center, Massachusetts Institute of Technology): We've got two cameras. This one's running at 20,000 f.p.s. This one's down at a mere 4,000 f.p.s.
DAVID POGUE: Yeah, and, for point of comparison, normal television like this that you're seeing right now is 30 f.p.s. So these are running a good bit faster.
First up, a piece of steel. It provides about as much protection as a 15th century breastplate.
Jim Bales: Three, two, one.
DAVID POGUE: Even this small caliber bullet easily penetrates, leaving a jagged hole.
Looks like someone has ripped a hole through a piece of paper.
To provide enough protection for a soldier's torso, this steel would have to be 10 times thicker. It would weigh an unwieldy 100 pounds.
What about a lighter weight material, like aluminum?
We see it mostly as flimsy rolls of foil but some forms of aluminum are just as strong as steel and are widely used in aircraft construction. Could aluminum serve as armor?
Lo and behold, there's a hole in the metal. Metallurgist, Mark Eberhart:
Mark Eberhart: This is beautiful. The energy of the bullet or much of the energy went into folding that metal back.
DAVID POGUE: The aluminum shows even more tearing at the point of impact. Pieces of metal light enough to be worn as armor aren't tough enough to stand up to the focused energy of a gunshot. When a bullet strikes the surface, the energy of impact is transferred to the metal in the form of heat, which causes the atoms to slide past each other.
Because the energy is focused on such a small area, the movement can be severe, causing cracks to form. If the material is thin enough, the bullet tears right through, like a hot knife through butter. So wearing a full metal jacket into combat is not a wise idea.
But if guns made personal armor obsolete, what are these guys wearing? It looks like cloth, but it's stronger than steel, and it's become standard equipment for soldiers, swat teams and bomb disposal squads.
I'm talking about Kevlar. When this synthetic fiber is woven into a fabric, Kevlar is strong enough to stop a bullet, or a blade, or even, with a few chemical changes,...
Ha ha.
...a fire.
Oh, it's hot, although, not as hot as he is.
Tucker Norton (Ballistics Scientist, Dupont): David, what you're about to see here are all the examples that we've got of Kevlar.
DAVID POGUE: This is Tucker Norton, ballistics expert and my tour guide here at DuPont, the company that invented the stuff.
Tucker Norton: Over here, we have some examples of vests where Kevlar is being used.
DAVID POGUE: Can I try one of these on?
Tucker Norton: Absolutely.
DAVID POGUE: Okay, I'm not here to show off the spring collection but to understand what makes this stuff so strong.
Kevlar, so important to American soldiers today, has its roots in a time when the entire United States was in peril. In the early '30s, the Japanese empire seized control of the world's silk supply, a material used to make parachutes and flak jackets. A frantic search began for a manmade replacement. Then in 1935, Wallace Carothers, a brilliant chemist at DuPont, created nylon, the first fiber made in a test tube, not in nature.
His breakthrough ushered in the modern age of synthetics. Then, in the 1960s, Stephanie Kwolek, a chemist, also at DuPont, created Kevlar. It remains the strongest synthetic fiber ever produced.
I know Kevlar can hold up in the most extreme punishment, but I want to see it up close. Our target: a block of clay designed to simulate the human torso.
It's protected by several layers of Kevlar. On the other end: the weapon, or rather the "bullet delivery device."
This is the gun?
Tucker Norton: This is the gun.
DAVID POGUE: Oh, I was expecting a "gun."
Tucker Norton: No, none of that.
DAVID POGUE: Oh, you're kidding?
What's going to happen? Someone's going to pull the trigger and... is there a trigger?
Tucker Norton: So the trigger is located outside of here. We don't actually do anything firing here, while we're inside.
DAVID POGUE: You guys are these giant safety wusses.
Tucker Norton: Now, here's where we go to fire.
DAVID POGUE: I feel like the President, failsafe.
Okay, ready, aim, fire!
Tucker Norton: You did it.
DAVID POGUE: Geez, I am so good.
Time to inspect the damage. Even with Kevlar, the simulated chest takes a beating.
Whoa! This is...this would have been my chest?
Turns out it looks worse than it is.
Tucker Norton: Unlike the human body, this clay will hold its position. The human body will respond and go back to normal.
DAVID POGUE: So, on a human, this would just be a really nasty bruise?
Tucker Norton: Yes.
DAVID POGUE: And maybe a broken rib?
Tucker Norton: That's right.
DAVID POGUE: Gotcha.
Tucker Norton: That's right.
DAVID POGUE: Still, ouch! But where's the bullet?
In fact, it's in the bag.
Tucker Norton: The bullet's still in here.
DAVID POGUE: Oh, my gosh. Can we see it?
Tucker Norton: Absolutely. So we have many, many layers, of course. The first one, second one, and usually about the third layer is where it gets stopped. And there it is. There's the bullet.
DAVID POGUE: Oh, my gosh.
It took just four layers of Kevlar, each less than a millimeter thick, to stop the bullet. The seven other sheets kept that hole from getting any deeper.
Tucker Norton: And as deep as any type of trauma might look, it's much better than that bullet going all the way through you.
DAVID POGUE: Right, I guess I could agree with that.
Kevlar is clearly some tough stuff.
Whoa!
But how does it work?
Tucker Norton: If you were to zoom down at about 100-million times...
DAVID POGUE: Well, we have a really nice camera, can you zoom down 100-million times?
Kevlar is a polymer, a long repeating chain of atoms, in this case, carbon, hydrogen, oxygen and nitrogen. Each chain is like a stiff piece of spaghetti. Gathered into bundles, the stiff chains create a thread-like fiber that has a hard surface, extremely high tensile strength and enough toughness to absorb the impact of a bullet.
Stopping a round is impressive, but there are other threats out there. How does Kevlar stand up to a knife or an ice pick?
Don't try this at home.
I'm not going to pay a lot for this muffler!
I said, "Caesar, on the side."
Twenty cents a text message? Are you nuts?
That's amazing. How can it do that?
Tucker Norton: Well, it does it because of the tight weave and because of the strength and durability of Kevlar. The tight weave prevents the spike from getting all the way through, from working its way through. And the strength of that Kevlar actually helps blunt that tip and maybe even bend that tip if you are strong enough.
DAVID POGUE: Since it was invented, Kevlar has been used in all kinds of products, from tires to parachutes and even cables. And that gives me an idea.
Tucker, I've got this friend. Um he's got a fleet of nuclear aircraft carriers and, they have these steel cables, a lot like this, that are designed to stop aircraft that are landing, 130 or 150 miles an hour. How does the strength of one of these cables compare with a steel cable?
Tucker Norton: Well, we'd expect the strength of this, the tensile strength, to be actually equal or better than something like the steel cable.
DAVID POGUE: And, it's lighter and it's less dangerous if it snaps?
Tucker Norton: That's right.
DAVID POGUE: Tucker doesn't think that anyone has ever tested Kevlar arresting cables, but I think I may be on to something.
This, this could be a sales opportunity for you.
Tucker Norton: We appreciate that, thank you very much, David.
DAVID POGUE: I'll look for my commission.
With its tensile strength greater than steel, extreme flexibility and heat resistance, in a package that weighs one-fifth as much as steel, Kevlar could, one day, be a replacement for steel cables. But already there may be other, stronger alternatives.
Scientists like Ray Baughman, at the University of Texas, are exploring the remarkable properties of a brand new material.
I'm talking about the carbon nanotube.
Ray Baughman (Chemist, University of Texas at Dallas, NanoTech Institute): Dave, this is one type of carbon nanotube.
DAVID POGUE: This is what it's all about, right here?
Ray Baughman: This is what it's all about.
DAVID POGUE: You know, I had a hunch they'd be like this. I just thought they'd be smaller.
I'm right. They're about a billion times smaller. Nanotubes are made of carbon atoms arranged in a rolled up, chicken-wire like structure. Besides nanotubes there are other forms of pure carbon, like diamond and graphite, the stuff of pencil lead. Nanotubes get their strength from the extremely strong bonds between carbon atoms.
Ray Baughman: These carbon nanotubes can have spectacular mechanical properties, spectacular strength and very high toughness.
DAVID POGUE: And you make them here in this very lab?
Ray Baughman: Yes, we do.
DAVID POGUE: Ah, so this is where it all happens.
Ray Baughman: This is where the process begins.
DAVID POGUE: And guess what? It's not even that difficult. Here's all you need:
One, a smooth surface with a thin layer of catalyst, a chemical that helps jump-start the tubes; two, a special mix of gases, full of carbon atoms, and three, this furnace, which, by the way, is really, really hot.
How much heat is it in there?
Mírcio Dias Lima (University of Texas at Dallas, NanoTech Institute): Right now, it's around 800 Celsius.
DAVID POGUE: That's about 1,500 degrees Fahrenheit.
Wow, man, I could nuke a pizza in like five seconds in there.
Mírcio Dias Lima: Yeah.
You can open more. More. See the nanotubes?
DAVID POGUE: Oh, man, that is blasting heat. Oh, I see the little black wafer in there. That's it?
Mírcio Dias Lima: Yup.
DAVID POGUE: That's nanotubes?
Mírcio Dias Lima: Those are, yes, exactly.
DAVID POGUE: Wow. That is very cool, baking away in there. Wow, it's like a nuclear tanning bed.
After just a few hours, the wafer is covered with a thick, black fuzz.
So, it looks just like a piece of black velvet.
So, if I were to really look in close to that, what would I see? What's it really look like under the microscope?
Ray Baughman: Well, we call this array of carbon nanotubes a nanotube "forest."
DAVID POGUE: The name is apt. Each nanotube looks like a tall and thin bamboo tree: hollow inside, so thin that if you could scale one up until it was one inch wide at the base, the top of it would reach two miles into the sky. Now, picture nanotubes by the billions, all standing shoulder to shoulder and you have one of Ray's nanotube forests.
Ray Baughman: There are, in fact, there are about 200 billion of carbon nanotubes in that area.
DAVID POGUE: Hold on one second, I'm going to count them.
Actually, I shouldn't be touching these. Scientists don't yet know if they are toxic.
Even so, there is already a rapidly-growing market for nanotubes particles as strength additives for tennis rackets, bicycle frames, even high-end car bumpers. Many products already incorporate carbon fibers, which are part carbon, part plastic. But nanotubes have greater tensile strength and toughness. Ray has set his sights on making super-strong materials that are 100 percent pure nanotube, made from his nanotube forests.
Ray Baughman: These aren't just ordinary carbon nanotube forests. They are nanotube forests that have a very special type of connectivity between nanotubes.
When we pull out one nanotube, that nanotube pulls out its neighbors, who pulls out other neighbors, to self-assemble a yarn or a sheet.
DAVID POGUE: Expert nano-yarn spinner Chi Lewis Azad gives me a hands-on lesson.
Chi Lewis Azad (University of Texas at Dallas, NanoTech Institute): Would you like to try?
DAVID POGUE: This I recognize.
First, we start with a forest on a wafer.
Chi Lewis Azad: You see, you can just grab on to this little orange tab. Now, don't make it too high of an angle, and make sure it doesn't touch the edge, and just pull out.
DAVID POGUE: Fast or slow?
Chi Lewis Azad: Whichever you feel comfortable with.
DAVID POGUE: Oh, dude!
As I pull on this little tab, nanotubes are coming off of the wafer, hooking other nanotubes and pulling them off of the slide.
Chi Lewis Azad: Try faster.
DAVID POGUE: I'm so good at this.
Chi
Lewis Azad: At too high of an angle it
will—as this is now
—it will start to break off from the forest.
DAVID POGUE: It's like coming out forever. I'll see you at the Texas county line!
It's still going, it's still going.
Chi Lewis Azad: It's still...There, it broke.
And, what's even neater, I can show you right here, I can even do a little
twist.
DAVID POGUE: Oh, you're making...
Chi Lewis Azad: Make it into a yarn right here.
DAVID POGUE: Insta-yarn.
Chi Lewis Azad: Look at that.
DAVID POGUE: Wow.
Chi Lewis Azad: It's about 1/100th of a human hair.
DAVID POGUE: Oh, my gosh. It is like steel. I'm pulling really hard. You probably can't even see that. In fact, look at this. It's so fine, you can't even see it.
Oh, that helps.
Chi Lewis Azad: Uh hunh. Keep twisting, keep twisting.
DAVID POGUE: Keep twisting? Okay.
It's amazing!
Chi Lewis Azad: It's stronger than steel.
DAVID POGUE: Stronger than steel? So why then, don't you take a bunch of these, twist them together, and make this super-cable that would be like bridge suspension cables and aircraft carrier cables?
Chi Lewis Azad: Can you imagine spinning a bunch of these all day long, to make into a rope that's a foot in diameter?
DAVID POGUE: Okay, so go the other way. Make the aircraft carriers really, really small.
Chi does have a point, for now. But in the future, materials we make may be strong, light and cheap enough to build incredible structures: a bridge across an ocean, suspended by cables made of fibers like Kevlar or nanotubes; a geodesic dome over an entire city, its structure made of carbon.
And in the future, we may not merely make these materials, we may grow them. That's what Mother Nature has been doing for hundreds of millions of years, engineering strength into the bodies of animals, one atom at a time.
Meet Marc Meyers. He's been fascinated by nature's engineering tricks ever since a boyhood encounter in a Brazilian forest.
Marc Meyers: My father was hunting, and I saw this entire toucan skeleton. Then I picked up the beak and I was amazed at how light it was, and how strong it was.
DAVID POGUE: The memory stayed with him for 30 years, until he happened to stumble across, of all things, a toucan farm. There, he made a startling discovery. In the toucan's amazing beak, nature has constructed a large, lightweight and amazingly tough structure. He wanted to know how.
I'd like to know why. Conservationist Joan Embery is on hand to explain.
What is the point of this huge beak? I mean, it does look a little ridiculous.
Joan Embery (Embery Institute for Wildlife Conservation): Well, they're primarily a fruit-eater, and that extends their reach. They use it as a screwdriver. They use it to find food. Also works defensively as a dagger. The edges are serrated.
DAVID POGUE: You tell me that now, when the thing is like six inches from my eye?
Joan Embery: That's why you're holding the tame toucan.
DAVID POGUE: Oh!
When Marc analyzed the beak of the dead bird, he found that it was made out of two different materials, neither one of which is strong.
On the outside is the shell. It's like bendable plastic, it feels like a, like a fingernail.
Marc Meyers: If the beak was just like this, it would flex. It would, it couldn't grab anything.
DAVID POGUE: The inside of the shell is filled with bone, but bone that's so full of air pockets it's as weak as Styrofoam.
Marc Meyers: See how it can break easy? You see?
DAVID POGUE: Right. Nothing.
Marc Meyers: And, oh, you should try, too.
DAVID POGUE: Yeah, exactly. Well, it's, it's foam, so it snaps. Well it, it would snap if I had any upper body strength.
The thin shell and the foamy bone, neither one is tough , but when combined...
Marc Meyers: You can see that this is the foam.
DAVID POGUE: Mm-hmm.
Marc Meyers: And then we have the shell.
DAVID POGUE: Right. Each by itself...
Marc Meyers: ...is weak.
DAVID POGUE: ...is weak.
Marc Meyers: I can show...yeah. If you pull out...it can bend this, here, very easily. Here, you see?
DAVID POGUE: Yeah.
Marc Meyers: And, uh...
DAVID POGUE: And this I could snap, if it weren't an important teaching tool for you?
Marc Meyers: That's right.
DAVID POGUE: Yeah. And if you combine them...
Marc Meyers: And now, if you take and try to bend these two together, see?
DAVID POGUE: Oh, wow.
Marc Meyers: Yeah.
DAVID POGUE: It's like super-lightweight concrete. It feels...
Marc Meyers: Right.
DAVID POGUE: It feels like nothing.
Marc Meyers: It is strong, and it is, uh, it is very lightweight.
DAVID POGUE: Marc was amazed that evolution had solved such a difficult engineering problem. So he began looking for other examples of animal engineering, which soon led him from the jungle to the beach.
This is your lab? You work in a wetsuit on a table by the ocean?
Marc Meyers: For today it is. I've been, ah, studying, actually, these shells, the abalone, for some years, because they are very, very strong.
DAVID POGUE: The abalone has evolved to have a very tough shell which protects it from the pounding surf and from predators like sea otters.
What is this shell made of? It's not...you're saying it's not like other shells?
Marc Meyers: It's calcium carbonate, which is the same as chalk.
DAVID POGUE: Chalk? Huh.
Marc Meyers: But it's much, much stronger than chalk.
DAVID POGUE: Yeah, chalk is not especially strong. I notice, by pure coincidence, there's a piece of chalk right here. And I don't think of chalk as sea-otter-proof. I mean, it just crumbles.
Marc Meyers: It crumbles.
DAVID POGUE: The fact that it pulls apart so easily shows that chalk has very little tensile strength or toughness. But that's not the end of the story.
Marc tells me not to be fooled by chalk's apparent weakness, because it's actually a very strong material.
I was never any good at dominos.
Marc Meyers: Now, you said that this is weak, right?
DAVID POGUE: Yeah.
Marc Meyers: You broke it with your, uh...
DAVID POGUE: My bare hands.
Marc Meyers: Yeah. And now I'll show to you that you can put your entire weight here, if you step carefully, here.
DAVID POGUE: Use you as a ladder?
Marc Meyers: Yeah.
DAVID POGUE: Alright.
Marc Meyers: Put more in the middle.
DAVID POGUE: More in the middle?
Marc Meyers: Yeah, yeah.
DAVID POGUE: You've got to be kidding me. This is going to shatter 'em like glass.
Marc Meyers: See?
DAVID POGUE: Wow! One-hundred-eighty-five pounds of pure muscle!
Marc Meyers: Yes.
DAVID POGUE: Look at that!
What's going on here?
Chalk can withstand tremendous pressure. It's called compressive strength, and it's a property of ceramics, the stuff of pottery, bricks and the cement in concrete. But ceramics also have a weakness.
Bend them, drop them, shoot them or even take a hammer to them...
For your $25 pledge, we'll send you this handsome coffee mug.
...and they shatter.
As with other materials, the strength or weakness of ceramics is determined by how their atoms bind together. Chalk is made of calcium, carbon and oxygen atoms, which are bonded together tightly, with no wiggle room. They can't slide past each other. That lets them to stand up to tremendous pressure. But if a small crack manages to open a space between the atoms, it can quickly spread. That's why materials like chalk, even though they have high compressive strength, often seem fragile.
Take glass, another fragile material. Marc insists that it also has high compressive strength, even more than chalk. Frankly, that seems hard to believe.
Okay, here we go. I'm going to wind up with an ankle full of glass.
Oh, my gosh! The chalk is one thing, but these little skinny wineglass stems are something else entirely.
Marc Meyers: Now are you willing to try it with three or two?
DAVID POGUE: No.
Three glasses! Three glasses! Where's the Cirque de Soleil agent when you need him?
Marc Meyers: That's amazing! You did it!
DAVID POGUE: Alright, Marc, explain this in science terms, quick!
Marc Meyers: Ceramics and glasses, when we compress them, they're very, very strong, stronger than metals.
DAVID POGUE: Okay, shall we try one?
Marc Meyers: Yes go for it!
DAVID POGUE: Let's do one glass. One glass, people!
Marc Meyers: Let's do it!
DAVID POGUE: Whoa! Whoa! Oh, my god! I can't believe it! How is this possible?
Marc Meyers: You did it, Dave.
DAVID POGUE: One glass! I'm amazing!
Marc Meyers: It's the world record.
DAVID POGUE: Oh, my gosh! One glass, people! One glass! Watch quickly!
While that is impressive, the abalone shell blows glass and chalk out of the water, so to speak.
Like the toucan shell, it combines two materials to make its fortress of strength and protection. Its shell is 95 percent calcium, carbon and oxygen, just like the chalk, but it doesn't have the same brittle weakness. It's not fragile.
You're saying this is 95 percent the same as this, but this...
Marc Meyers: Yeah, but, try to break this.
DAVID POGUE: But this one...really?
Marc Meyers: Yeah, go ahead.
DAVID POGUE: This would cost a fortune at the gift shop.
Marc Meyers: No, yeah, go ahead. I dare you.
DAVID POGUE: Okay, if I were a sea otter, I would be a terrible failure. It's completely... Nope.
The shell demonstrates both compressive strength and toughness, the ability to absorb energy without breaking, thanks to the way that the abalone has engineered its protective cover on a microscopic scale.
As it constructs its shell, the abalone gathers calcium, carbon and oxygen from the environment to build rows of tiny, hexagonal plates. Each plate is brittle, but between the layers, the abalone inserts a protein, a long biological chain. That small addition acts like a shock absorber between the plates. The resulting shell is far less brittle, much tougher and more crack-resistant than simple chalk.
As we learn to apply the lessons of microscopic structure, engineers may one day build pressure-resistant ceramics and glass from the atom up, beautiful buildings of sculpted concrete that will never crack and will last 1,000 years, or a deep sea city with a spectacular view.
But when it comes to strong, light and flexible materials, built atom by atom, another natural material may hold the key.
It's not new. In fact, it's been around for hundreds of millions of years: spider silk. Spider silk has been shown to have more tensile strength than steel and Kevlar. It can stretch to 140 percent of its length without breaking and remains flexible, even in extreme cold. It's also so lightweight that a mere pound of the stuff could form a single strand long enough to stretch around the equator.
But could we harvest enough to put it to use?
I paid a visit to the American Museum of Natural History, in New York, where I met Nicholas Godley.
This is it? This is your baby?
Nicholas Godley (Fashion Designer): This is the big one.
DAVID POGUE: ...who enlisted the help of more than a million spiders to make this breathtaking piece of fabric.
Nicholas Godley: It's the largest known textile and sample of piece of spider silk in the world.
DAVID POGUE: To truly appreciate this remarkable material, you have to feel it.
The main piece is so valuable it's off-limits, even to its creator, but Nicholas has brought a smaller sample.
It feels really, really, really soft, like animal wool or something.
Nicholas Godley: I challenge you to break off this piece.
DAVID POGUE: I'm going to break before it does. Okay, it does not...it's like pulling a strand of steel. Wow!
It's beautiful and it's super-strong. Could we ever put spider silk to practical use?
Nicholas Godley: It's very difficult to do this on a commercial scale. It took a million-sixty-three-thousand spiders, roughly, to make...
DAVID POGUE: Geez!
Nicholas Godley: It takes about 20 minutes for each spider, and they produce about 400 yards of thread.
DAVID POGUE: Each thread was pulled, by hand, from a spider's spinneret.
It took four years and millions of strands to weave this 11 foot-long masterpiece. Its rarity is a testament to the sheer difficulty in harvesting this material. But that may soon change, thanks to this guy.
Randy Lewis (Molecular Biologist, University of Wyoming): So here's where we keep our spiders. Um, we have special little cages for them, in this room.
DAVID POGUE: Spider central, huh? Whoa, not funny. Not funny!
Randy Lewis: You're now an official Spiderman affiliate!
DAVID POGUE: This is Randy Lewis, a biologist at the University of Wyoming, who's stuck on spider silk.
Wow.
Randy Lewis: This is a golden orb weaver.
DAVID POGUE: Golden orb weaver?
RANDY LEWIS: Just cup your hand and get behind her.
DAVID POGUE: Why is she making a web right now?
RANDY LEWIS: Because she wants to make sure if she falls, she catches herself.
DAVID POGUE: Oh, so she's constantly spinning out that dragline.
RANDY LEWIS: Whenever they move, they leave the dragline behind. That's kind of where it got its name.
DAVID POGUE: Randy has been fascinated by the amazing properties of dragline silk for fifteen years.
And he knows all too well the difficulty of extracting silk from spiders. So he set out find a way to mass produce the stuff, in hopes of revolutionizing the world of strong materials.
Wyoming is ranch country, so when Randy began to consider how to solve the problem, his thoughts turned to livestock. He figured maybe he could combine a little old-fashioned animal husbandry with the emerging science of genetic engineering.
Now, thanks to Randy, these goats have just a little bit of spider in them.
"Transgenic," that's the word for what these goats are.
RANDY LEWIS: Right. It means that they have a gene from another organism that's been put into their chromosomes.
DAVID POGUE: Genes are sections of D.N.A. that contain the encoded instructions for making proteins.
Scientists identified the two genes in spider D.N.A. that make silk. They copied one of the genes and spliced it into the D.N.A. of goats so that they would make spider silk protein in their milk.
I'm a little worried about what we're going to find in here—eight-legged goats, giant dripping webs?
Randy Lewis: You won't be able to tell the normal goats from the transgenic goats, 'cause they're all exactly the same.
DAVID POGUE: Aww, I had a dog like you once.
It's impossible, just by looking, to tell these goats from their non-spidey brethren, but, scientifically, they're very valuable.
Yeah, you're special. You've been genetically modified. How does it feel to be genetically modified? Huh?
Only 50 percent of Randy's goats will inherit the necessary gene, and the females that are born with it will go on to produce spider silk protein in their milk.
And that's what I've come all this way to see for myself.
Can I... try it?
Randy Lewis: You're certainly welcome to. We normally use the electric, just because it's a whole lot faster, but, um, you're welcome to
DAVID POGUE: Faster? But you've never seen me milk a goat.
Randy Lewis: All right, well, you're about to find out here.
DAVID POGUE: So this one's skim and this is two percent?
RANDY LEWIS: Well, in this case, they're both spider silk. You can choose either one you want.
DAVID POGUE: Okay, so I just grab and tug, just like...?
Randy Lewis: Right, just squeeze down, and just use your fingers, and just...
DAVID POGUE: Wow.
What percent of this milk is spider-silk stuff?
RANDY LEWIS: For most of these, it's relatively low, so we're talking about... um... maybe one to two percent.
DAVID POGUE: Gives two percent milk a whole new meaning.
Randy Lewis: That's right, two percent, um yeah. We'd love, we'd love to see 10 percent, but we'll take two percent, at this point in time.
DAVID POGUE: Next begins the painstaking process of coaxing the silk protein out of the milk.
Randy Lewis: So we take the milk that we collected out at the farm, we pump that onto this column, which has a whole bunch of very small tubes in it.
DAVID POGUE: Right.
Randy Lewis: Tubes have holes in it, so that the spider silk protein and the milk proteins come out of the tubes, and it keeps the fat inside.
DAVID POGUE: So what's left is basically goat skim milk?
Randy Lewis: Exactly.
DAVID POGUE: Eventually, Randy comes away with a vial of highly concentrated silk protein.
How much milk went into making this tiny drop of solution?
RANDY LEWIS: Um, from our best goats that would be about a quart of milk.
DAVID POGUE: Wow. And then, what...how much silk can you get out of this?
Randy Lewis: We can get at least two to three meters.
DAVID POGUE: Wow! Alright let's have a look.
The final step is the most delicate. Randy's team takes the protein liquid and slowly injects it into an alcohol bath, which causes the liquid protein to solidify into a strand of actual spider silk.
So this is it? So this is your manmade spider silk?
Randy Lewis: This is manmade spider silk.
DAVID POGUE: Now, to prove its mettle, Randy loads the strand into a machine that measures tensile strength.
Randy Lewis: Look, you can start to see it moving.
DAVID POGUE: It is, it's straightening out.
The silk stretches as it resists being pulled until...
It just broke. I'm sorry, Randy.
Randy Lewis: It just broke.
DAVID POGUE: But there's nothing to be sorry about. A computer measures the force it takes to break the sample, telling them its tensile strength. Remember, the tensile strength of steel, its resistance to being pulled, is what kept my plane from plunging into the ocean.
The tensile strength of spider silk appears to be greater than steel or even Kevlar. Not as strong as carbon nanotubes, but more flexible, easier to make in long strands, and certainly not toxic.
Randy Lewis: We are stronger than Kevlar, we're stronger than steel, but we're not stronger than the natural silk.
DAVID POGUE: The spider silk protein demonstrates nature's ingenuity. Its ingenious structure is comprised of three distinct sections.
Randy compares one of those sections to children's building blocks.
Randy Lewis: If you look at a molecular model, what you see is that they have little pins and little holes, just like LEGOs. So if you take and put them together and stack them up, like this, and try to pull them apart...
DAVID POGUE: These are, these are the proteins here?
RANDY LEWIS: These are the proteins. And that's part of the protein we try to pull apart. It doesn't pull apart.
DAVID POGUE: Okay, so if I'm pulling this way and this way, and this is the dragline, and it's not coming apart...
Randy Lewis: Yeah, that's right. So that's one part of the dragline.
DAVID POGUE: Proteins: good for you. Okay.
Randy Lewis: So four hundred million years, spiders've been playing with LEGOs.
DAVID POGUE: The second section of the protein is stretchy.
Randy Lewis: For elasticity, they have something that literally looks like a molecular spring. When you pull on the ends...
DAVID POGUE: Right.
RANDY LEWIS: ...it stretches.
DAVID POGUE: Okay, so...
Randy Lewis: And, and, and that the elasticity...
DAVID POGUE: This would be a different protein?
RANDY LEWIS: No, it's the same protein. It has different parts.
DAVID POGUE: Okay.
Randy Lewis: So we have, in the same protein, we have LEGOs, ...
DAVID POGUE: Right.
Randy Lewis: ...we have springs,...
DAVID POGUE: Okay.
Randy Lewis: And then, in order to put them together, we have zippers.
Zippers hold things together very well, but they have some flexibility, so you have a way of being able to put this together. With a spring, might be too tight to do that, so you put a zipper in between: now you have dragline silk.
DAVID POGUE: These basic building blocks repeat over and over again, trillions of them in each strand of silk.
The complex structure is evolution's way of enhancing the strength of the common raw materials found everywhere in nature.
Before I leave, Randy suggests one last experiment for us would-be Spidermen.
You getting anything?
RANDY LEWIS: I don't feel a thing.
DAVID POGUE: Nothing.
So it seems that the next chapter in the story of strong materials is already upon us.
For thousands of years, we've experimented with our planet's raw materials to make metals and ceramics. Then, through chemistry, we created synthetics and built materials atom by atom. Now scientists are learning to combine the engineering tricks found in the living world with the incredible strength of our own inventions. With these new tools and materials, there's no telling what we'll be able to accomplish, once again propelling human civilization across a new frontier, as has happened again and again, over millennia, every time we learn more about making stuff stronger.
Broadcast Credits
Making Stuff: Stronger
- Host
- David Pogue
- Written, Produced and Directed by
- Chris Schmidt
- Executive Producers
- for Powderhouse Productions
- Joel Olicker
Tug Yourgrau - Co-Producer
- Alexandra McHale
- Field Producer
- Doug Gordon
- Edited by
- Brian Cassin
- Additional Editing
- Justin Vaida
Daniel McCabe
Kristine Gaffney - Director of Photography
- Gary Henoch
- Additional Photography
- Joe Livolsi
Jason Longo
Mike Marshall
Joe Friedman
Tom Kaufman - Sound Recordists
- Glen Ackers
Mark Arees
Lyle Bibler
Jeff Boedeker
Mike Boyle
Kabe Cornell
Gregg Hinnen
Mike Italiano
Dave Manahan
Bill Wander
Warren Wolfe - Grips and Gaffers
- Ken Perham
Greg Price
Grady Smith
Darrell Tawney
Josh Weinhaus - Production Assistant
- Daniel V. Parsons
- Music
- Lunch Special Music
- Animation
- Edgeworx, LLC
- Additional Animation
- Wlan Waldo
Jenny Olson - Production Manager
- Diane Knox
- Post Production Supervisor
- Kevin Young
Michael Fallon - Assistant Editors
- Eric P. Gulliver
Peter Hyzak
Bryce Patingre - Assistant Online Editor
- Erin Gallagher
- Online Editor / Colorist
- Julie Kahn
- Audio Mix
- Heart Punch Studio, Inc
- Research
- Sonia Weinhaus
- For Powderhouse Productions
- Post Production Manager
- Melissa Walter Richards
- Senior Production Coordinator
- Carlin Corrigan
- VP of Production
- Daniel Miller
- Senior VP of Production and Post
- Robert Kirwan
- Archival Material
- AP Archive
Getty Images
Footage courtesy of Discovery FootageSource
Footage Courtesy MIT Museum
Footage Provided by Thought Equity Motion
iStockphoto
Palmpress, Inc. - Special Thanks
- Producers gratefully acknowledge the cooperation of the Materials Research Society.
- Richard A. Souza
Amy Moll
Kristin Bennett
Jerry Floro
Megan Frary
Kevin Jones
Tommie Kelley
Alex King
Aditi Risbud
Stephen Streiffer
Rick Vinci
Sandra DeVincent Wolf - Special Thanks
- American Museum of Natural History in New York City
United States Navy
Otis Elevator Company
Professor Stephen Sass, Cornell University - NOVA Series Graphics
- yU + co.
- NOVA Theme Music
- Walter Werzowa
John Luker
Musikvergnuegen, Inc. - Additional NOVA Theme Music
- Ray Loring
Rob Morsberger - Post Production Online Editor
- Michael H. Amundson
- Closed Captioning
- The Caption Center
- Publicity
- Eileen Campion
Victoria Louie
Karen Laverty - Marketing
- Steve Sears
- Researcher
- Kate Becker
- NOVA Administrator
- Kristen Sommerhalter
- Production Coordinator
- Linda Callahan
- Paralegal
- Sarah Erlandson
- Talent Relations
- Scott Kardel, Esq.
Janice Flood - Legal Counsel
- Susan Rosen
- Post Production Assistant
- Darcy Forlenza
- Associate Producer Post Production
- Patrick Carey
- Post Production Supervisor
- Regina O'Toole
- Post Production Editor
- Rebecca Nieto
- Post Production Manager
- Nathan Gunner
- Compliance Manager
- Linzy Emery
- Development Producer
- Pamela Rosenstein
- Supervising Producer
- Stephen Sweigart
- Business and Production Manager
- Jonathan Loewald
- Senior Producer and Project Director, Margret & Hans Rey / Curious George Producer
- Lisa Mirowitz
- Coordinating Producer
- Laurie Cahalane
- Senior Science Editor
- Evan Hadingham
- Senior Series Producer
- Melanie Wallace
- Executive Producer
- Howard Swartz
- Managing Director
- Alan Ritsko
- Senior Executive Producer
- Paula S. Apsell
Produced by Powderhouse Productions
A NOVA Production by Powderhouse Productions for WGBH
© 2011 WGBH Educational Foundation
All rights reserved
Image
- (David Pogue)
- ©WGBH/Mark Ostow, ©iStockphoto.com/OGphoto
Participants
- C Lewis Azad
- NanoTech Institute, UTD
- Jim Bales
- Edgerton Center, MIT
- Ray Baughman
- The University of Texas at Dallas www.nanotech.utdallas.edu/personnel/staff/baughman.html
- Mark Eberhart
- Author, Why Things Break
- Joan Embery
- Embery Wildlife Conservation
- Nicholas Godley
- Fashion Designer
- Randy Lewis
- Molecular Biologist, UW
- Marcio Dias Lima
- NanoTech Institute, UTD
- Mark Meyers
- Materials Scientist, UCSD
- Tucker Norton
- Ballistics Scientist
- John Paules
- GM, Ellwood Technologies
- Scot Vannorman
- United States Navy
- Danny Womack
- Owner, Outlaw Motor Speedway
Education and Outreach Resources
Preview
Full Program | 53:54
Full program available for streaming through
Watch Online
Full program available
Soon